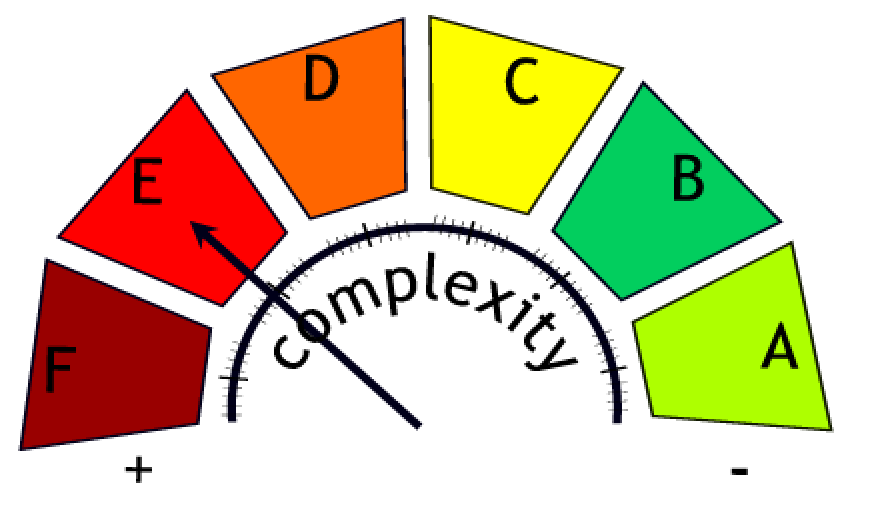
Respiration-controlled, semi-closed systems
Tekst by Jan Willem Bech
December 2022
After years of reading about rebreathers, a breath-controlled rebreather remains an intriguing system. It therefore took me a long time to understand the system in terms of concept. Most of the people I talked to about it did understand that they are semi-closed systems that thus expel gas and therefore create bubbles, but the real depth I unfortunately missed in many articles. This page makes an attempt to explain the concept to understand more of the system than using tables that come with the rebreather. I will try to explain the concept based on an early rebreather produced by Halcyon in the 1990s.
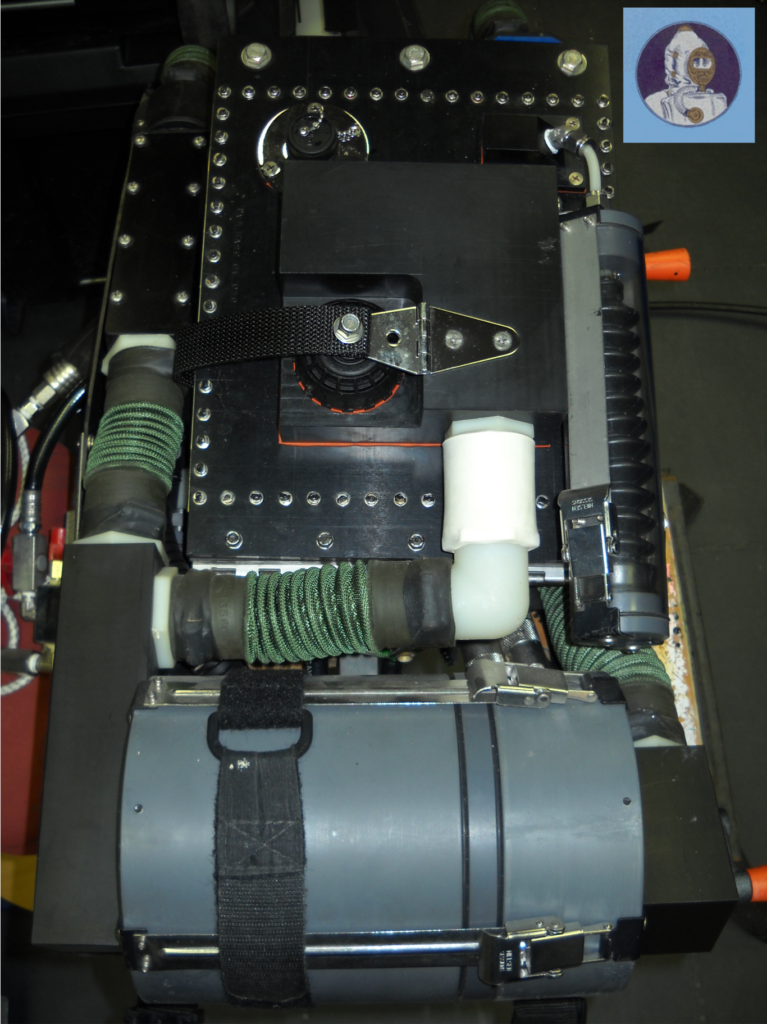
The name of the system is quite lengthy but does not say much more than that the system works passively, i.e. there are no automatic systems in it that add gas, that only happens when the diver breathes. The concept of Variable Ratio makes the story complex but so interesting. But let’s start at the beginning!
Almost 150 years ago (!), a Russian inventor Achilles de Khotinsky worked in New York and later moved to the Netherlands and settled in Rotterdam. He was the first to obtain a patent for a diving apparatus working with bellows. His patent can be found under US 244,062 and was registered in 1881.
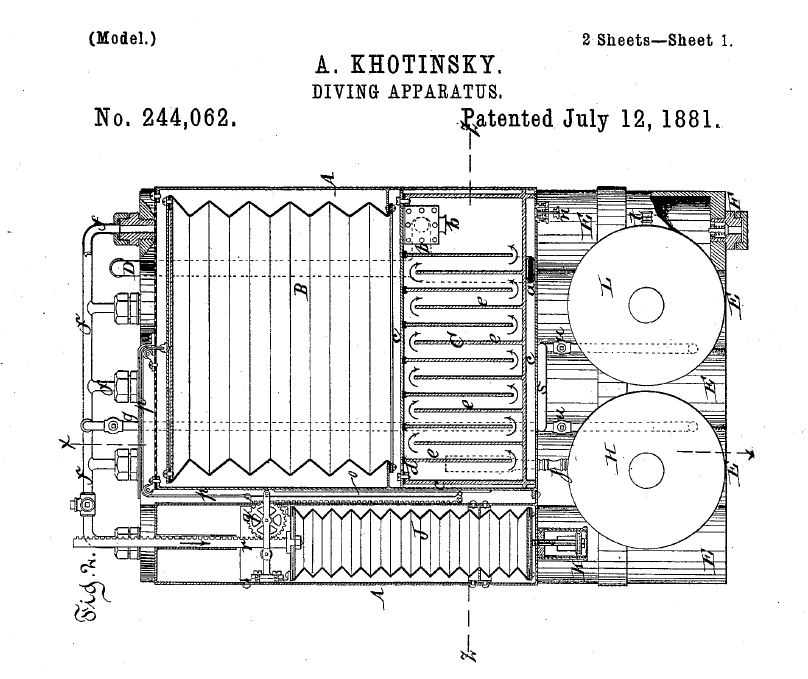
The draft
The vast majority of semi-closed breathing systems use a gas injection that works with a nozzle or restiction. This supplies a constant flow of gas to a breathing circuit. If the diver wants to be able to dive deeper than 6 metres, the injected gas will have to be diluted with a dilution gas. This dilution gas ensures that the oxygen partial pressure remains below about 1.6 bar. This 1.6 bar partial oxygen pressure is a limit chosen based on experiments for sport divers. Higher oxygen pressure can lead to hyperoxia, or oxygen toxicity. So if we were to dive with pure oxygen only, at a depth of 6 metres this partial pressure will be exceeded and the diver may drown. For this reason, we dilute the gas with a dilution gas. For most semi-closed systems with which we do not dive much deeper than 40 metres, we dilute the oxygen with nitrogen so we use a mixture known as Nitrox. This mixture is continuously injected into the breathing circuit. The diver only partially uses the oxygen in the gas, converting it to CO2. The nitrogen will accumulate due to the constant injection, creating an overpressure in the circuit at some point. The excess gas is now pushed out of the circuit via a pressure relief valve. The excess gas is released in the form of bubbles. The system described above is used to this day as an Active Semi Closed Rebreather system.
It took a long time to develop a system where this constant injection was no longer needed and gas was supplied only when the diver needed it. In other words, a passive semi-closed system. This was eventually achieved by using a mechanical transmission that transfers a movement resulting from human breathing to a metering valve. A logical consequence is that oxygen consumption is linked to breathing frequency. Something also had to be devised to remove gas, preferably also linked to breathing frequency. Here, the principle of the bellows was developed, in which a small bellows is compressed into a larger bellows. Each time the volume decreases due to breathing and oxygen consumption, a small dose will be blown out of the small bellows into the surrounding water and at the same time a small amount of fresh gas will be supplied.
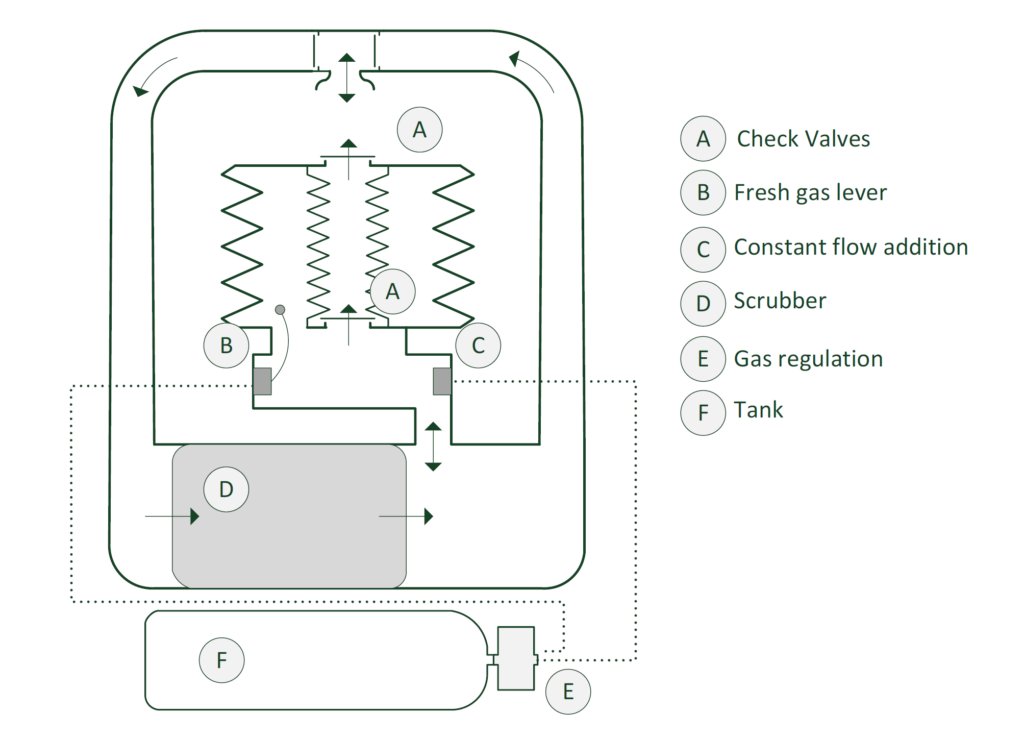
Ratio
To make the best use of the system, another problem presented itself. As the depth increased, the ambient pressure will increase. This will also increase the partial oxygen pressure of the breathing gas. As a result, the gas pushed out by the small bellows will start to contain more and more oxygen. As a result, gas consumption will increase unnecessarily and there will also be variable oxygen partial pressure. This is undesirable. The number that represents the volume ratio between the small and large bellows is a fixed value that causes this phenomenon. To make the system more efficient, this ratio should preferably be able to change. If, as we dive deeper, the amount of gas we discard becomes smaller, the efficiency of the system will increase considerably! This is where the concept of variable ratio comes in. Using a simplified diagram, we will take a closer look at how the system works!
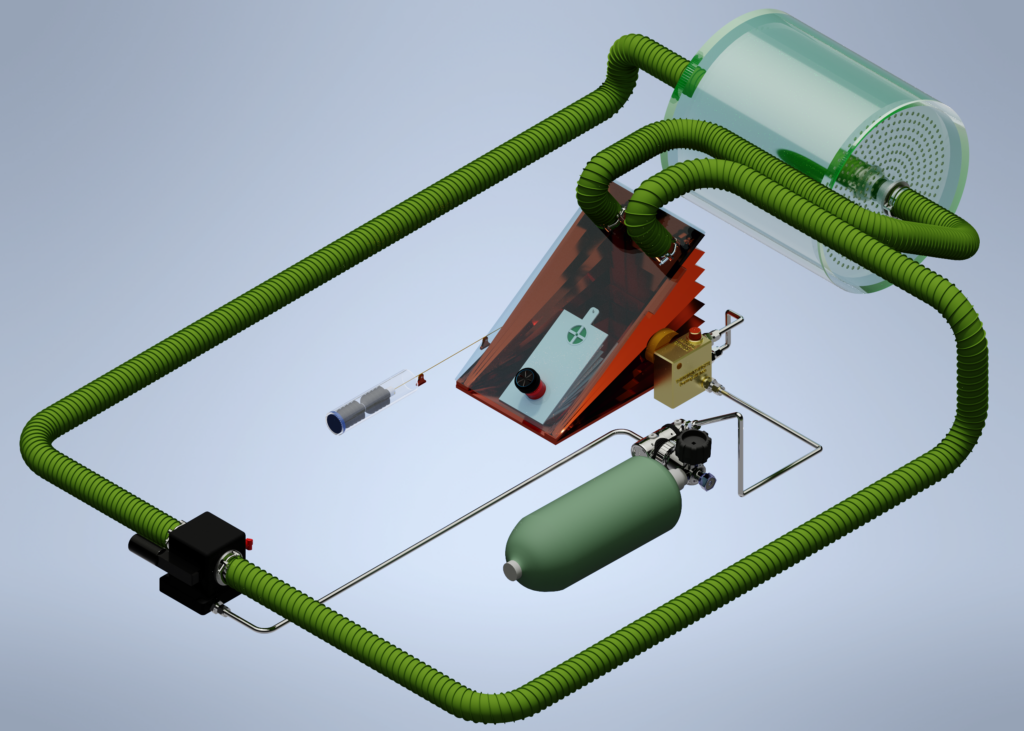
The above drawing shows a simplified diagram of a semi-closed system with a variable ratio mechanism.
The drawing below shows the main components involved in our system. In reality, there are more components and there are mechanisms that are not normally visible because they are, for example, inside the bellows.
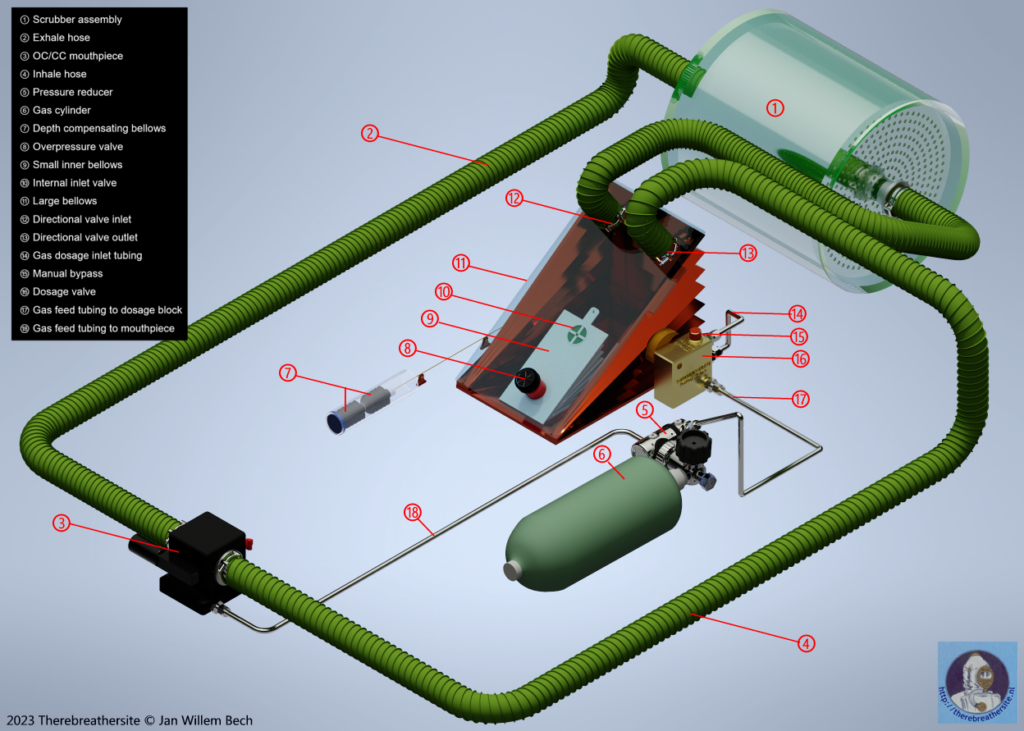
Gasaddition
The breathing gas must be controlled by the diver’s breathing. Several techniques have been developed for this purpose. In this example, I show a simplified representation of the technique in the drawing below. A characteristic of a passive system is that the breathing gas is only supplied when the volumetric volume (the amount of remaining gas) has decreased to such an extent that a mechanical system operates a valve or restrictor. This valve will inject gas for a short time until the mechanism releases the valve and stops the gas supply. The system shown has a large bellows where the breathing gas is stored outside the diver’s lungs. In this case, in the large bellows A. Think of this as the counter lung in which the clean gas from the scrubber is supplied. The new breathing gas is also premixed here so that the diver is always offered oxygen-rich gas through the breathing hose 4.
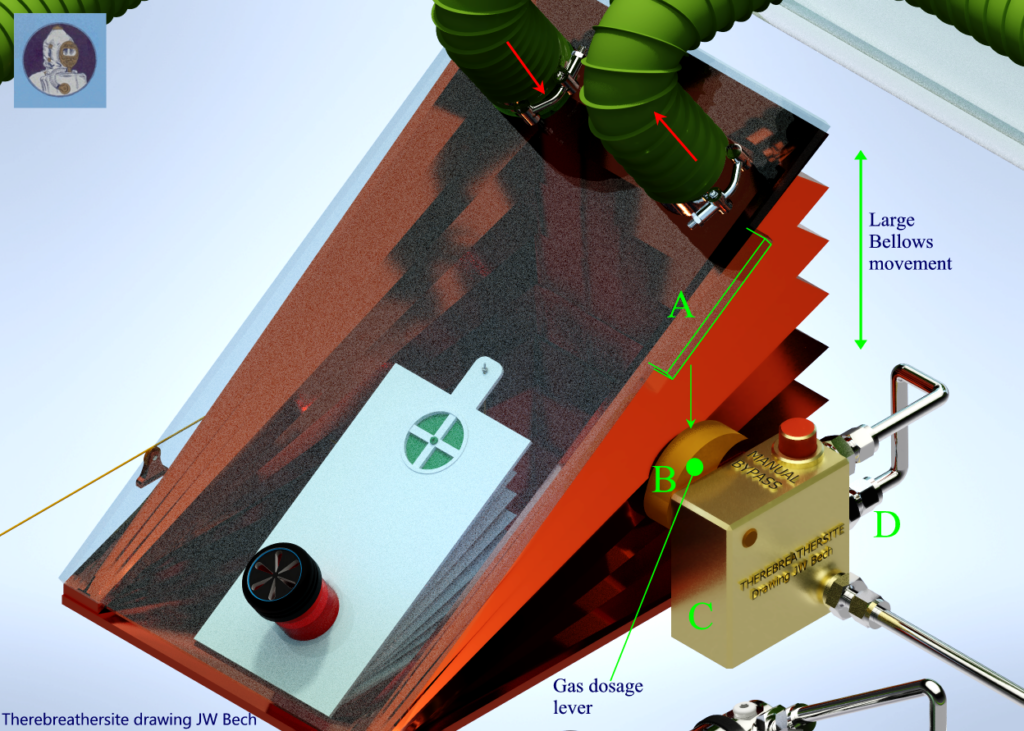
The large bellows contracts as soon as the diver inhales and expands as soon as the diver exhales. For this explanation, I have drawn the metering valve (C) outside the bellows and also provided a manual bypass button. In reality, this valve will be located inside the bellows and the bypass button will be positioned in a place easily accessible to the diver and will inject the gas directly into the breathing circuit.
Once the diver inhales, the protruding cam (A) will move down through the contracting bellows and depress the gas dosage lever (B). This will cause fresh gas to flow into the large Bellows through pipe (D). the breathing gas contained in the bellows will be enriched by the new oxygen-rich fresh gas.
Ditching the gas
As described earlier, the diver will only consume the oxygen in the gas mixture. The carbon dioxide produced by the diver will be chemically bound by the carbon dioxide absorbing granules in the scrubber. After the breathing gas has passed through the scrubber, the deoxygenated gas will enter the large bellows where it will be replenished with oxygenated gas by the dosing system . Should this process continue, a surplus of nitrogen is created and must leave the system. The gas will therefore not only be in the large bellows, but will also find its way out through the internal valve in the small bellows and the overpressure valve on top of it, which is connected to the surrounding water. This valve is adjusted so that the compression of the large bellows also depresses the small bellows and forces the gas out of the overpressure valve. The illustration below shows how the gas is mixed at the bellows but also squeezed out of the system. Fresh gas or oxygen-rich gas injection takes place on the inhalation side of the barrel.
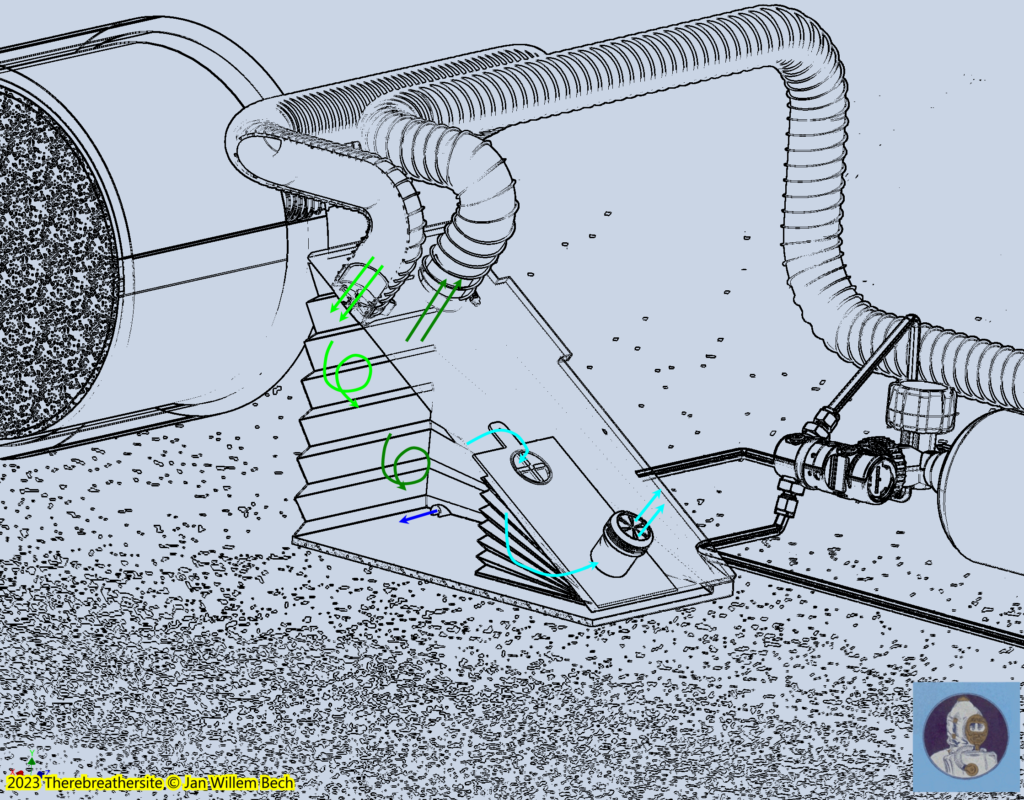
Depth compensation, the brains of this machine
To use the system as efficiently and deeply as possible, it is important to keep the partial oxygen pressure within limits. We know from active semi-closed systems that the fraction of oxygen inhaled by the diver is lower than the fraction of gas supplied. However, the partial pressure will increase with the depth of the dive! For regular active semi-closed systems, the fraction can be calculated as follows:
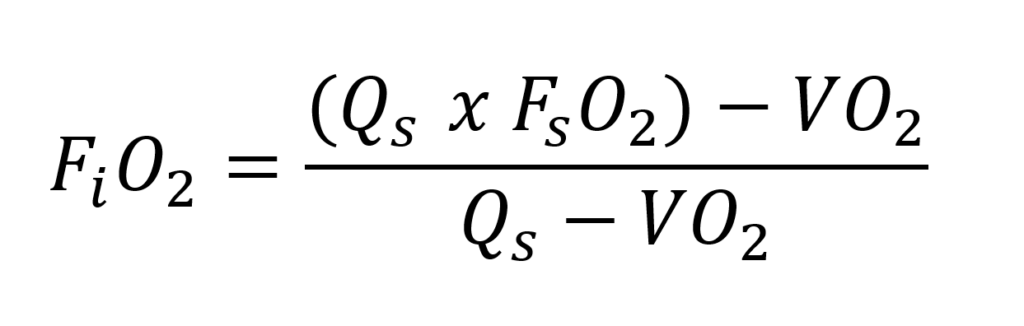
- FiO2 = inhaled fraction by the diver (%oxygen/100)
- Qs = The volume of breathing gas that is being injected continuously (L/min)
- VO2 = The volume of the diver’s metabolised oxygen (l/min)
- FSO2 = The O2 fraction in the injected breathing gas (% oxygen/100)
If, in an active semi-closed system, we inject nitrox with 50% oxygen at 7.3 litres per minute and the diver metabolises o.9 l oxygen per minute will result:

This calculation shows that the gas inhaled by the diver at a depth of 10 metres at a water pressure of 2 bar has a partial oxygen pressure of 0.86 bar. At 30 metres, the partial pressure has already risen to 4 bar x 0.43 = 1.72 bar. This clearly reaches the depth limit at which this active system can be used for diving.
The passive system makes it possible to mix the inhaled oxygen fraction as close as possible to the fraction of injected gas. The principle behind this is the unique property that by the deeper we dive with the passive system, less gas is released to the environment. The so-called depth compensation system is also called variable ratio because the volume ratio between the large and small bellows in the system changes with depth! Let’s see how one managed to do that.
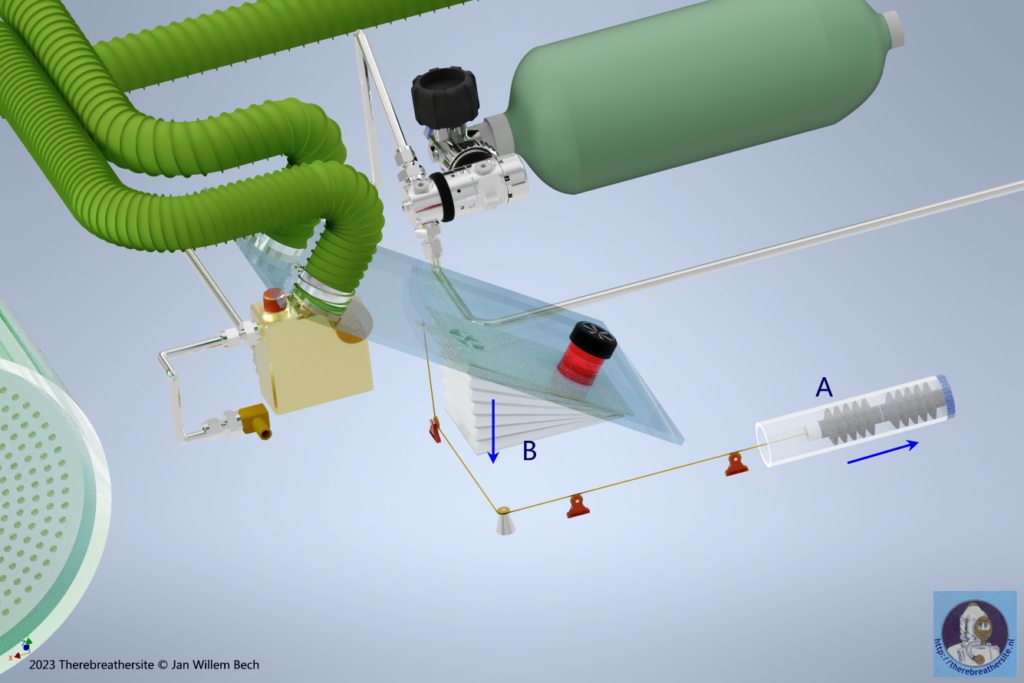
In the drawing above, you can see the depth sensors in the form of two axially coupled bellows. As soon as the diver descends, the water pressure will depress the air-filled bellows and, via the cable, the small bellows will partially shrink, reducing their volume. This changes the ratio of the volumes of the small and large bellows. The ratio factor increases. As a result, less gas will be dumped into the environment at depth. This will allow the diver to breathe longer from the large bellows before new gas will be added. This conceptual principle requires some imagination but can be further investigated with a calculation. The result is that the oxygen fraction is optimally regulated in relation to the injected gas and varies over the entire dive spectrum between 7% at the surface and 2% at depth. Moreover, due to this mechanism, independent of labour (metabolism) this fraction will remain almost the same while only gas consumption will increase with labour.
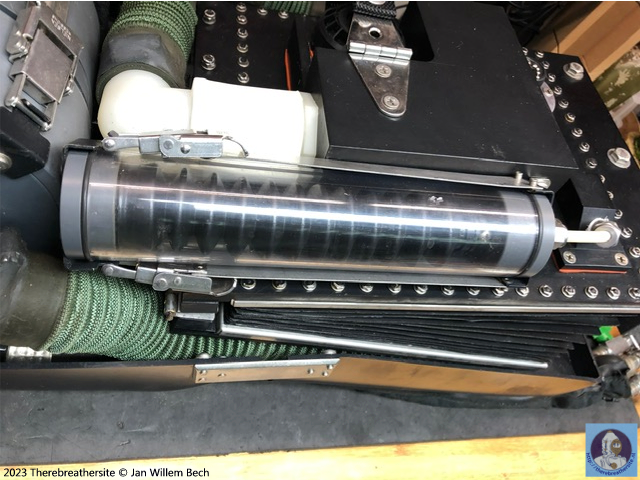
To explain this mathematically, there are first some new values that come into play in calculations with this passive variable ratio system.
There is a relationship between metabolic consumption and a diver’s breathing rate. As soon as more work has to be done, the body needs more oxygen. The amount of oxygen depends on many factors including physique, fitness and, above all, the work to be done. The volume a diver exhales per minute is called the respiratory minute volume (RMV) and is denoted by VE. We refer to the diver’s metabolic consumption as VO2. Dividing these factors by each other, we calculate the Ventilatory equivalent of oxygen also called the K factor (in some calculations referred to as Ke ). This unitless constant has a value for divers between 14 and 28. The low number obviously represents the trained fit diver. (Ref: 1957 Lanphier & Camporesi; Respiration and Exertion).
An example of the calculation is: A diver breathes a tidal volume of 2.5 l/min, he has a breathing frequency of 25 breaths per minute. His oxygen consumption is 3.47 l/min because of this
K = (2.5 l/min x 25)/3.47 = 18
Ve/VO2 = K (1)
Another factor is the factor showing the relation between of the variable dosage of the exhaust below(Vd) and the exhaled gas by the diver (Ve), both in ltr/min. This ratio factor R (in other calculation reffered as Kd)
Vd/Ve = Rd (2)
We can now work through some known parameters that must be known for a calculation to calculate the inhaled oxygen fraction. For this, the following data must be known:
D = diving depth in mtrs (3)
EANx = composition of the supply gas (4)
FsO2 = fraction oxygen of the supply gas (5)
VO2 = metabolic consumption of oxygen (6)
We can now establish the so-called steady state formula for this type of rebreather. It should be noted that a special feature of the rebreather in our example is the variable exhaust volume (the PVR in the name) which makes Rd vary with depth (after all, the volume of the small bellows changes with depth). The formula applicable to this type is:
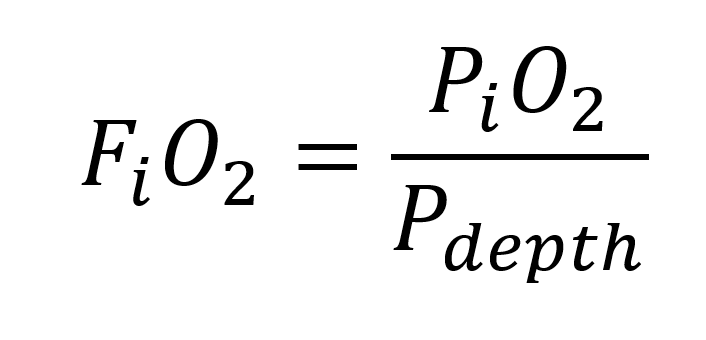
Above the inhaled oxygen fraction equation (7)
The the final steady state formula is as follows:
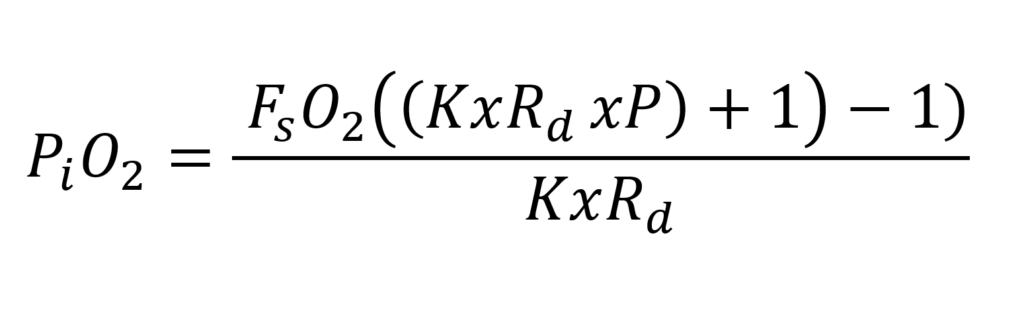
Above the steady state formula for the PVR-BASC rebreather (8)
Let’s make an example calculation to compare the variation in the inhaled fraction at different depths from 0 – 30 metres.
Our diver decides to make a dive to 30 metres depth using EAN 32 (nitrox 32% O2).
He dives with a Halcyon PVR-BASC with a ratio of 0.7. His metabolic consumption is 1.5 ltr per minute. During the dive, he breathes 15 times per minute and his breathing volume (tidal) is 2.5 litres. We can now establish the following:
- P = 1 bar at 0 mtrs, 2 bar at 10 mtrs, 3 bar at 20 mtrs and 4 bar at 30 mtrs
- Rd = 0,7 this is the value for this rebreather
- K = Ve/VO2 = (2,5 l x15 b/min )/1,5 l/min = 25
Putting this in the formula at 0 meters gives a FiO2 is 0,2811
Putting this in the formula at 10 meters gives a FiO2 is 0,300
Putting this in the formula at 20 meters gives a FiO2 is 0,307
Putting this in the formula at 30 meters gives a FiO2 is 0,310
The variabele in oxygen fraction is minimal!
If we change the metabolix rate VO2 as a result of hard work @ 30 mtrs and change it from 0,75 – 1,0 – 1,5 – 2 and 3:
With a metabolic rate of 0,75 @ 30 mtrs de FiO2 is 0,315
With a metabolic rate of 1,0 @ 30 mtrs de FiO2 is 0,313
With a metabolic rate of 1,5 @ 30 mtrs de FiO2 is 0,310
With a metabolic rate of 2,0 @ 30 mtrs de FiO2 is 0,307
With a metabolic rate of 3,0 @ 30 mtrs de FiO2 is 0,300
The change in the metabolic rate hardly affects the inhaled oxygen fraction
Realiteit versus theorie
The above system is mechanically extremely complex. It requires great skill in maintenance and is fragile during transport. Besides these disadvantages, the risk of hypoxia also plays a role. If the bellows fail, the diver can become unconscious without noticing due to a lack of oxygen in the mix. Besides these disadvantages, the original variable-ratio rebreather is generally so large and heavy that more modern (electronic) systems are often chosen. They also often switch to a system where depth compensation is not present as in the Halcyon RB80 or similar systems. These constant volume systems can be mechanically constructed much more simply by using two round bellows (one large and one small).
In this example, the bellows system is placed after the scrubber and in front of the nozzle. The injection of the fresh gas can also take place at different positions. The model used in this article is purely to explain the principle. However, producing such a rebreather still raises many complex issues. For instance, the Halcyon rebreather is equipped with a water disposal system, the bellows for depth compensation are duplicated and the gas injection is designed in a different way. Imagine also the challenge of attaching a cable to the small bellows where it must be able to move through the wall of the large bellows and be gas-tight and liquid-tight! That the system is more complex shows the schematic representation of the system below:
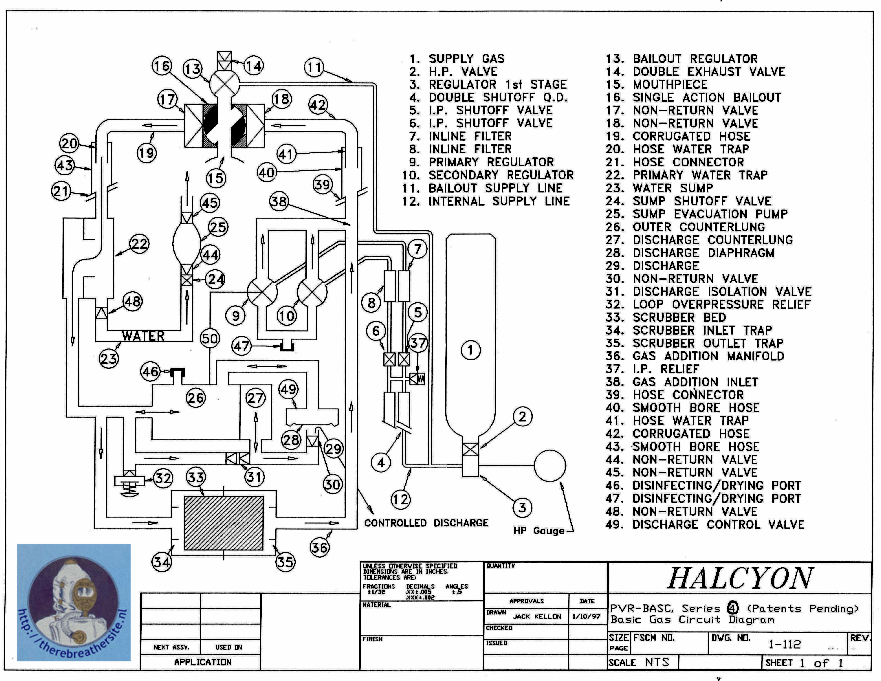
More Systems
Semi-enclosed systems can be classified into different versions. Unfortunately, there are no uniform names for the systems and they are therefore used interchangeably. We distinguish between:
- Active rebreathers based on constant injection or constant mass flow using premixed gas
- Active rebreathers based on constant injection or constant mass flow using two gases mixed through the system
- Passive systems using a demand principle and emitting a constant volume of gas
- Passive systems using a demand principle and emitting a constant mass of gas
- Passive systems using a demand principle using two gases mixed through the system
This classification also has hybrid variants such as the DC55 (Fenzy) or which can use a constant mass flow system in addition to a demand system for shallow diving. (The CMF system is supplied as a separate part).
The system in the example uses bellows. Another system such as the IS-Mix or AGA ACSC uses a dosing chamber in which the fresh gas is stored. Breath-controlled valves open the valves sequentially, allowing gas to flow out of the dosing chamber into the barrel on exhalation and filling the dosing chamber on inhalation.
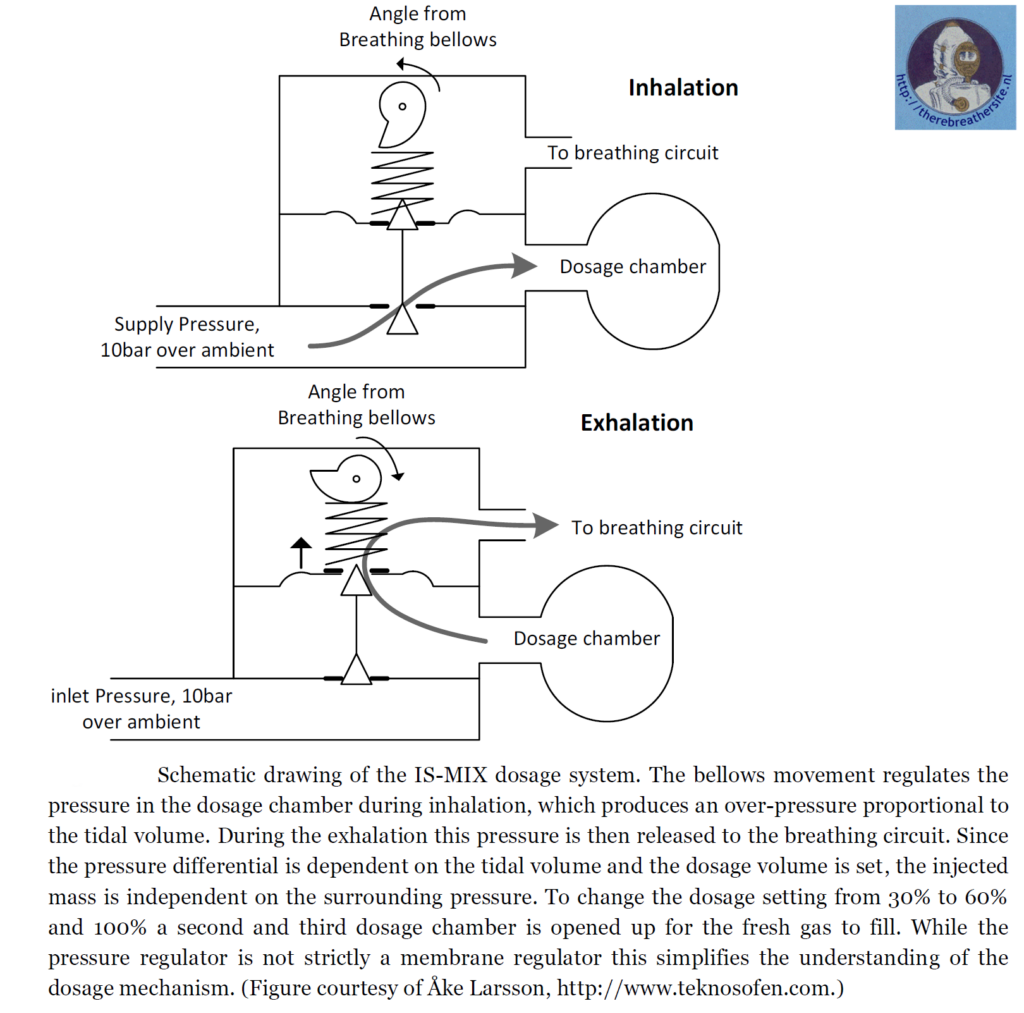
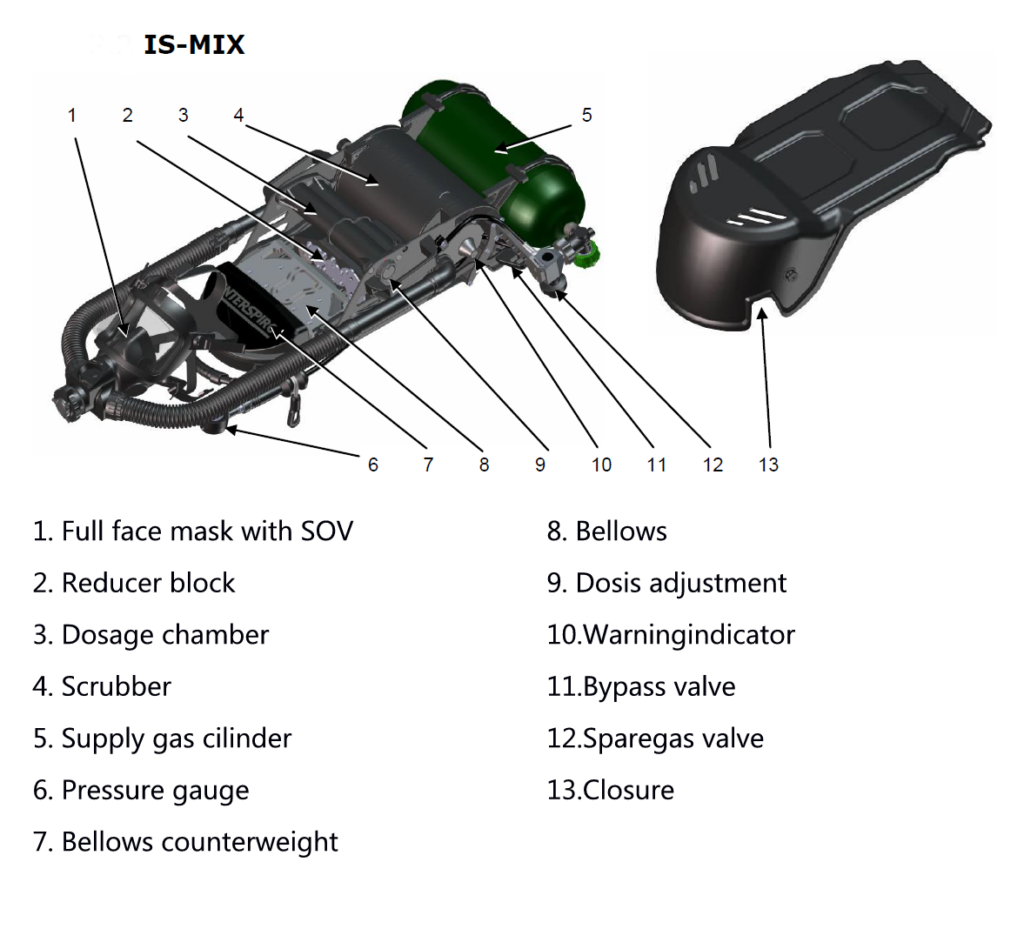
In 2015, Dr Oskar Frånberg, Associate Professor Oskar Frånberg, Associate Professor
of Lunds universitet Department of clinical sciences published his thesis with the topic:
Oxygen content in semi-closed rebreathing apparatuses for underwater use. Measurements and modelling.
This paper contains unique information about the background of passive rebreather technology. I am therefore very pleased with Dr Frånberg’s permission to publish his thesis here! Much appreciated!

Therebreathersite was founded by Jan Willem Bech in 1999. After a diving career of many years, he decided to start technical diving in 1999. He immediately noticed that at that time there was almost no website that contained the history of closed breathing systems. The start for the website led to a huge collection that offered about 1,300 pages of information until 2019. In 2019, a fresh start was made with the website now freely available online for everyone. Therebreathersite is a source of information for divers, researchers, technicians and students. I hope you enjoy browsing the content!