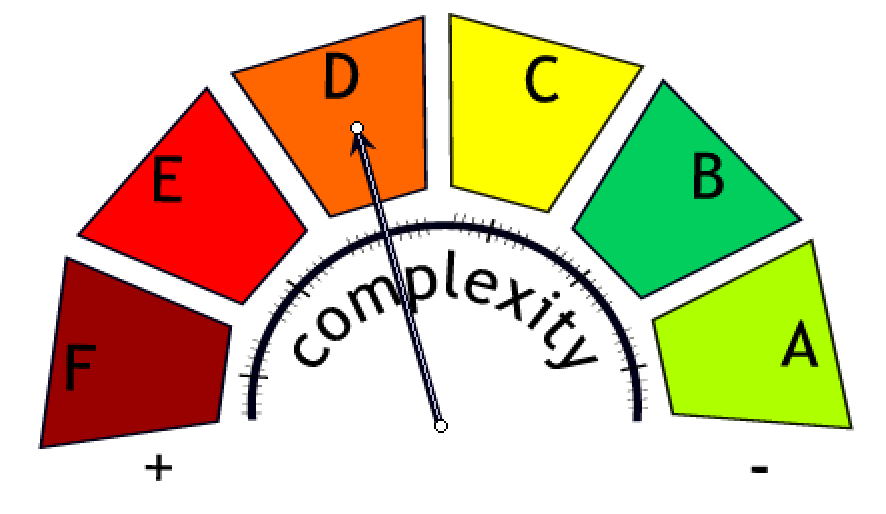
Diving systems and oxygen fire
Rebreathers and oxygen fires
In technical diving, we use enriched but also degraded gases. As a result, technical divers will face the risk of oxygen fire. This risk is generally underestimated because, fortunately, oxygen fires are rare. Unfortunately, when such a situation occurs, it often results in casualties or serious injuries. Many divers are told in their training that oxygen must be handled with care, but real in-depth knowledge is often sadly lacking. I therefore recommend taking a blender training course and, if you want to gain even more knowledge, an oxygen service technician training course. On this page, I tip a few areas of focus, but they do not compare to the knowledge you gain by attending a training course!
When does the risk of an oxygen fire occur?
The risk occurs in an environment with increased oxygen concentration or oxygen pressure. This will usually occur in a system such as a diving cylinder, regulator or booster. Examples of such situations are:
- – Opening a cylinder filled with oxygen
- – Blending nitrox or trimix
- – Compressing enriched gases (continuous flow blending) with a compressor
- – Working with oxygen and a high pressure booster
- – Cleaning parts in contact with oxygen
- – Pressurising gas systems
- – Oxygen leaks through O-rings
- – Fitting a pressure reducing valve to a large cylinder
- – Using enriched gases in a pressure chamber
In all these cases, the special properties of oxygen will have to be taken into account. Oxygen is not a flammable gas; it is colourless and odourless. It has a strong catalytic function and is an essential part of a burning process. Fire is possible if three conditions are met: These conditions consist of the presence of fuel, the presence of oxygen and, as the last third condition, an ignition source.
Since all plastics and metals can burn in oxygen (!) provided the concentration and pressure are high enough, any oxygen system therefore contains 2 of the three elements to start a fire. What is missing is an ignition source. Ignition sources come in numerous varieties. However, it is important to understand that oxygen and pressure are the two most important variables.
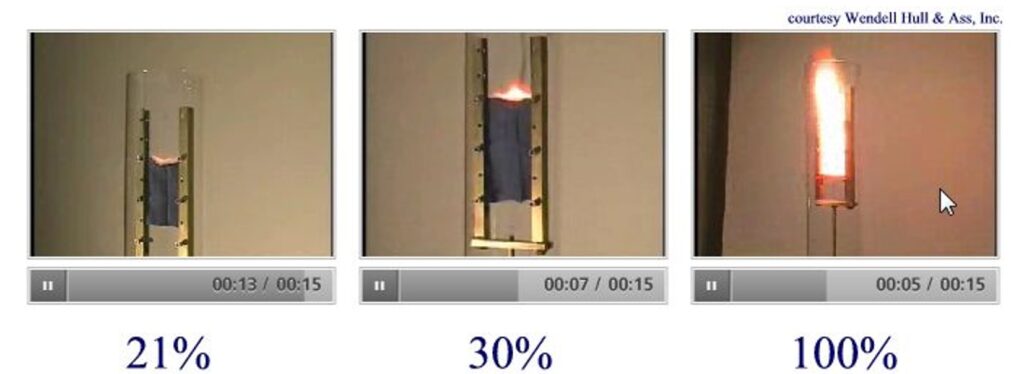
Here you can see the effect of oxygen in an enriched environment. Smoulder a clothing piece in 21%, at equal pressure, a strong stinging flame will occur at a higher concentration.
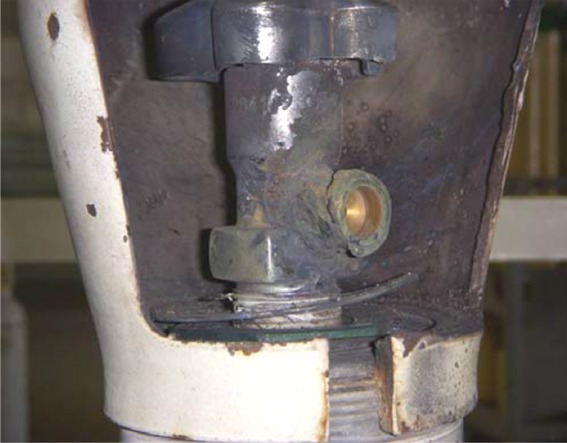
However, high pressure will further increase the risk, and in the event of a fire, metal will therefore feed the fire! Metals do have a sensitivity range where brass is the least susceptible to fire but will certainly be able to burn even at high pressure.
In diving, the most common oxygen fires are caused by three mechanisms:
- Adiabatic compression
- Particle Impact
- Contaminant Promoted Ignition
Let’s take a closer look at these three mechanisms:
Adiabatic compression:
Oxygen fires are often caused by adiabatic compression. As a result of a rapid increase in pressure by, for example, opening a tap too quickly on a filler panel, the oxygen in a pipe is compressed very quickly. The gas molecules rub along each other and the temperature increases. If this happens quickly, the temperature can rise very quickly and is capable of igniting non-metallic seals. If this process occurs so quickly that the energy (heat) developed cannot be released to the environment, a phenomenon called adiabatic compression occurs.
The temperature increase can be so extreme that this process causes the onset of an oxygen fire. After all, there is oxygen – ignition (fire) – fuel. After ignition, a chain reaction will occur under the influence of the oxygen present. The burning seal (for example: PTFE, rubber or viton) will burn very reactively under the influence of the high oxygen pressure and will be able to ignite metals. As the burning plastic burns extremely reactively with the oxygen under high pressure, the surrounding metal will melt and also burn.
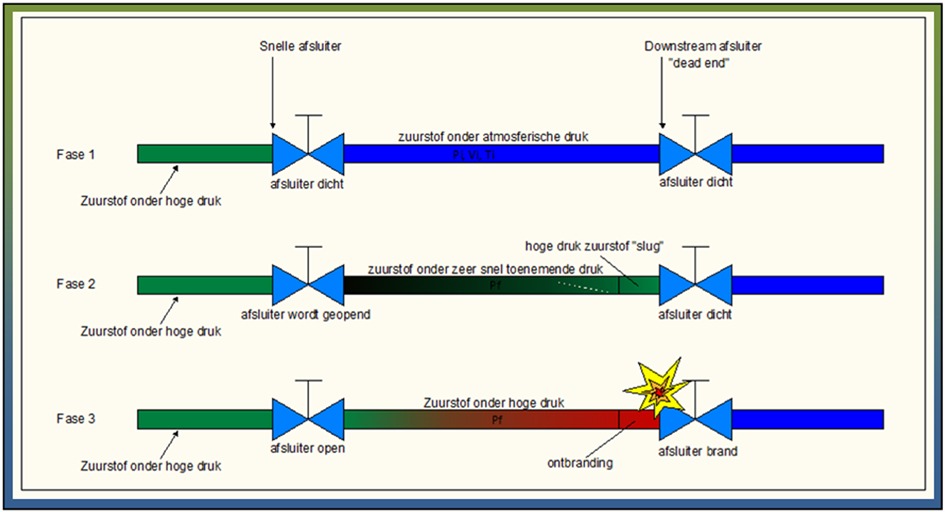
Phase 1: oxygen held back under high pressure by fast valve, lower pressure until dead end
Phase 2: Valve opens rapidly, pressure increases very rapidly
Phase 3 Due to extremely rapid pressure increase, ignition occurs on the dead end side
In general, it can be said that the pressure increase should take place within one second for small high-pressure installations and within several seconds for larger industrial installations. To calculate the temperature that can occur after opening the tap, here is an example: if we consider a room with a volume of 1 litre at 27°C and at 1 bar ambient pressure, which we suddenly expose to a pressure of 230 bar, the volume of 1 litre will be compressed while a large heat is developed. A few conventions are needed for this calculation example.
We calculate with ideal gases, i.e. we do not take into account behavioural changes of gases at different temperatures and pressures. We act as if the gas behaves constantly. Oxygen atoms can move in 5 directions, 3 rotation directions and 2 vibration directions. To calculate the temperature increase and the compression ratio, we need a special number called the adiabatic index, which we denote by the letter γ. Now, to determine the adiabatic index, we need to determine α. This factor is calculated by dividing the number of degrees of freedom of the O2 atom by 2. So α = 5/2
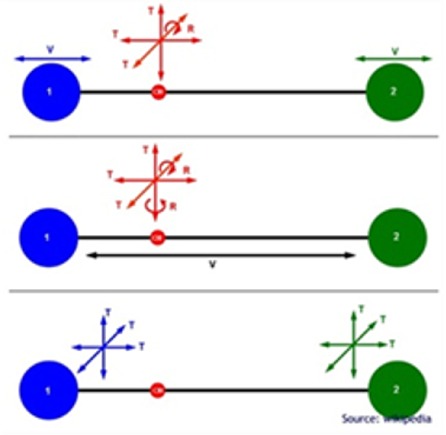
It is also true that:
We can then calculate that
So the adiabatic index for oxygen is 1.4. Now, it does depend on temperature and varies between -181°C = 1.450 and 400°C = 1.394, but at room temperature γ = 1.4. For our calculation example, we will use 1.4.
If we compress a volume of 1 litre to 230 bar, it seems that the volume of 1000 cc is reduced by the factor 230. Unfortunately, this is not the case. As the pressure increases, the temperature will also increase and thus the pressure will increase proportionally, the pressure increase is not linear! The term compression ratio was created for this phenomenon. The compression ratio can be calculated using the following formula:
We write the pressure in pascals. 1 bar = 100,000 pascals. We describe the volume in this case in cc. 1 litre = 1000 cc. In our example, the gas in the space of 1 litre is compressed to 230 bar. We now want to calculate what volume the gas occupies after being compressed. We calculate as follows:
So increasing the pressure to 230 bar only decreases the volume by about 50x!
So now calculate the temperature increase, we assume a temperature of 27 °C. This corresponds to 300 K. The ambient pressure is 1 bar = 100,000 Pa.
We use this constant to calculate the final temperature. After compression:
As a result of compression to 230 bar, the temperature increases from 27°C to 1147°C! It may be clear that there are particularly many materials that already ignite at lower temperatures! These extreme temperatures are practically not usually achieved. This is because the gas quickly gives off heat to its surroundings. However, the temperature increase can rise well above the ignition temperature of Teflon (450°C – 500°C) (Berthelemy & Vagnard, 1988).
Effect of adiabatic compression:
Compression of a gas under high pressure within one second can make the gas so hot that the materials used ignite. The high oxygen pressure can cause a fire that ignites non-metals and then ignites metal, developing a reaction chain. Prevention: Pressurise the system slowly. Use valves that can only be opened slowly. Hand-operated ball valves are not suitable for oxygen systems or for mixing gases! Use needle valves that can be opened with several turns.
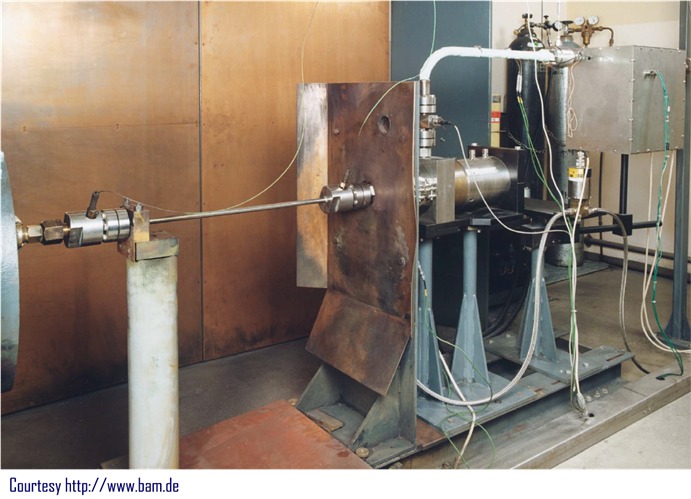
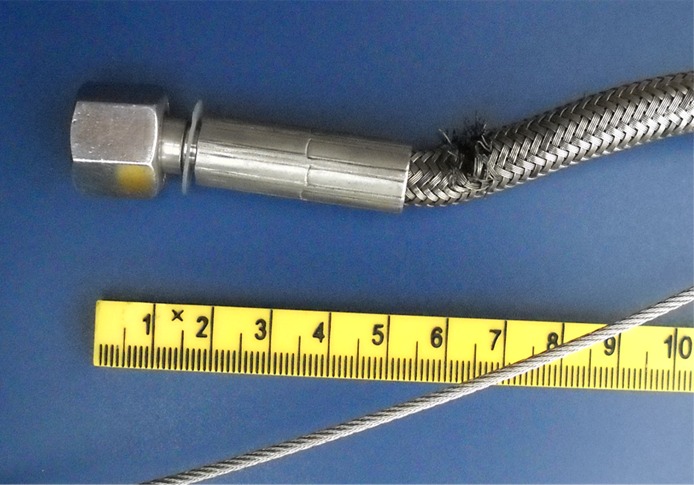
Particle Impact
Particle Impact could be translated as ‘ignition by particle collision’. However, since the term is also established in Dutch as Particle Impact, I use the term Particle Impact (PI) here. PI can act as ignition for an oxygen fire because loose small particles collide with metal parts at high speed. Ignition occurs because the particle itself ignites, or because the metal it collides with ignites. For such ignition to occur, there must be a high velocity at which the particle collides. Ignition occurs by transfer of kinetic, thermal or chemical energy. Experiments (Benz, Williams, & Armstrong, 1986) show that ignition occurs when aluminium particles of 2000 μm impact aluminium and steel alloys at speeds above 244 m/s. If the same impact test is repeated with alloys having a very high nickel and copper content, no ignition occurs. This shows that the latter alloys provide protection against particle impact ignition. A mixture of iron powder with inert impurities does not ignite below 45 m/s. It does above this speed. In an ambient pressure of 20 to 300 bar, no consistent ignition behaviour appears (Benz, Williams, & Armstrong, 1986), so the effects occur in this pressure spectrum. Particle impact ignition thus occurs at velocities above 45 m/s. This velocity is already reached at a pressure difference of 1.5% across a valve. If the pressure difference increases to 2:1, a sonic velocity (~305 m/s) will be achieved when the valve is opened. The effectiveness of Particle Impact ignition is enhanced if the particle collides with surfaces at an angle to the gas flow. In industry, a radius of a bend to diameter ratio of 1.5 is often used to minimise the PI effect (for a 12 mm pipe, a bend should have a minimum radius of 18 mm). Particle impact ignition occurs only if both the particle and the metal being hit are flammable. Some metals, such as aluminium, can also be ignited by inert particles, such as dirt. Incidentally, it has been found from research (Forsyth & Stoltzfus, Ignition Resistance of Hard (Type III) Anodised Aluminium to Particle Impact, 1997) at the White Sands Test Facility that anodised aluminium 6061-T6 has a greater resistance to ignition compared to bare (oxidised) aluminium 6061-T6. For reason that there are at least 30 known accidents with aluminium pressure regulators for oxygen delivery, their use is not recommended. As a pressure difference over a component greater than 1.5% already leads to velocities above 45m/s, there is always the risk of Particle Impact in breathing gas filling installations. Materials should therefore be designed accordingly.
Effect of Particle Impact: The impact of particles on surfaces in the gas flow can cause these particles or the surface to ignite. Copper, nickel and monel alloys appear to be the least sensitive to PI, while aluminium and titanium are at the top of the sensitivity range.
Prevention: Select materials that are insensitive to PI. These are typically materials with a high tolerance to Particle Impact ignition. For a list of material types, see EIGA document 13/02 Appendix C. Prevent contaminants in the pipe by using sintering source filters, for example. For diving and medical technology applications, filters of 10 μm are recommended. For industrial applications, filters of 150 μm to 600 μm are used.
Contaminant Promoted Ignition
This form of ignition is actually nothing more than contaminants, grease or oil forming the basis for an oxygen fire. By heating another component, this heat can ignite the contaminant. The burning contaminant, together with the oxygen, causes non-metals and even metals to ignite. The original heat generation comes from another process such as adiabatic compression, or flow heat generated by friction. Thus, oil can be easily ignited by oxygen. The burning contaminants such as burning oil components form a first link in the fire chain. Next, oxygen fuels the fire, causing non-metals to melt and burn. The non-metals ignite the metals and thus the fire enlarges. There have been cases of oxygen fires caused by an insect in the valve of a high-pressure cylinder. When the valve was opened, the biomass formed the fuel. The increase in pressure provided the heat and the oxygen provided the oxidiser for the fire.
In 2004, a fatality occurred in America due to contamination of a filling shop. The investigation into the cause of this accident writes: “It is probable that the diveshop fillsystem was contaminated and that this hydrocarbon-based contaminant was migrating into the cylinder during the cylinder filling operation. Experience indicates that hydrocarbon contaminants are easily ignited due to even mild compression heating or flow dynamics”. The fire that started in the cylinder led to the aluminium igniting partly due to the high concentration of oxygen and the bottle losing its structural strength.
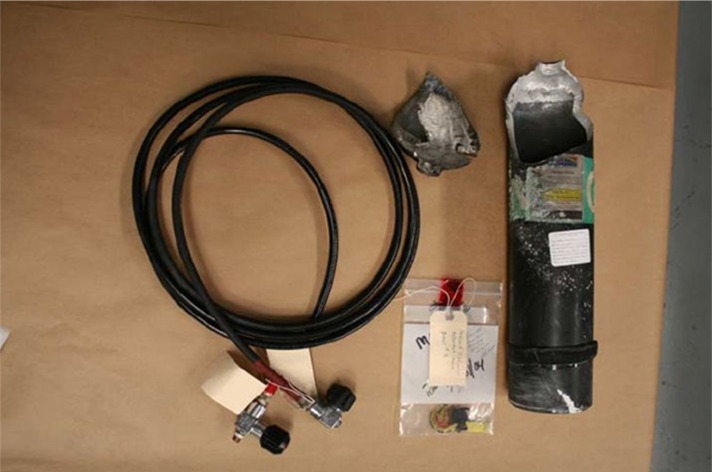
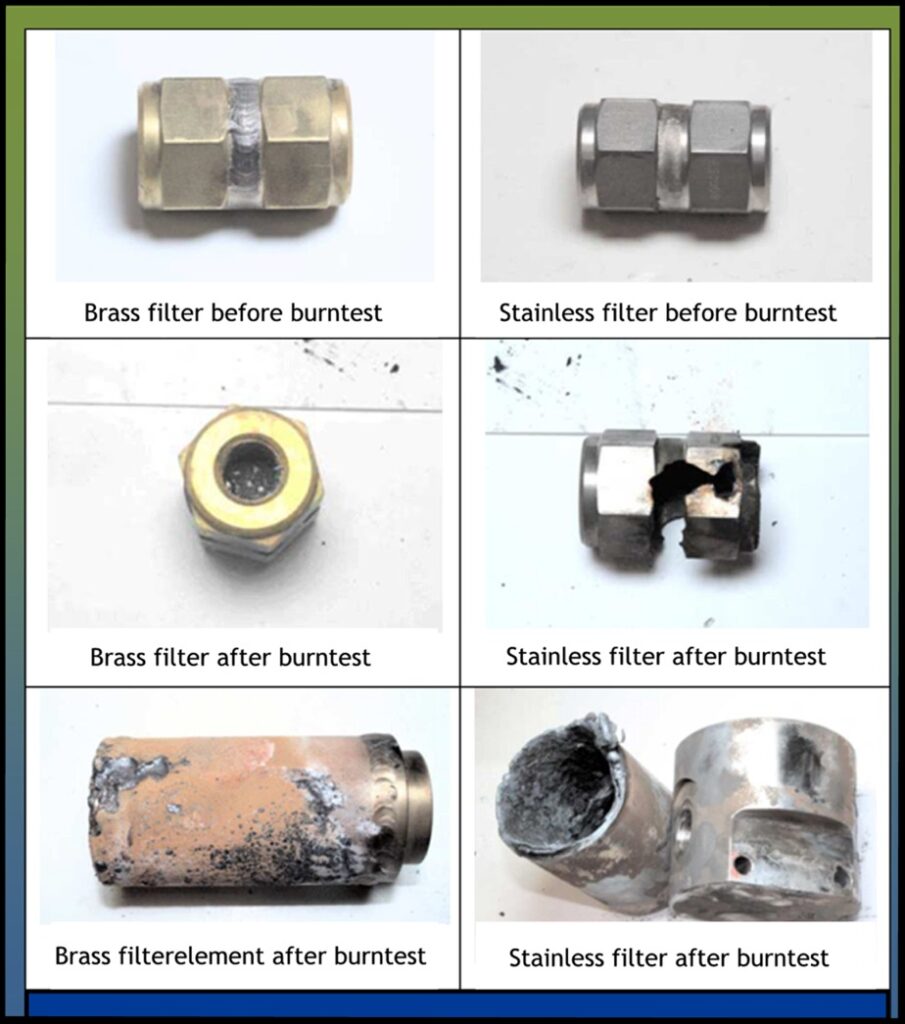
Effect of Contaminant-Promoted Ignition: Contaminants in, on or in the vicinity of the oxygen system can be heated up by other mechanisms and lead to ignition. It is a kind of indirect ignition. The burning non-metal will be able to ignite metals under the influence of the oxygen atmosphere, which then ignites the oxygen fire. In some cases as in the accident described above, the fire will be able to structurally destroy a pressure vessel.
Prevention: Make sure the system is free from contamination by grease, oil dirt particles wood paper etc.
Besides these three relatively common causes of oxygen fire,
we know of many special properties of oxygen fire:
- – Promoted ignition data, the degree of susceptibility of a material to ignition
- – Autogenous Ignition Temperature (AIT), at which temperature materials self-ignite
- – Limiting Oxygen Index, the resistance of a material to ignition (suitability)
- – Heat of Combustion, HoC, the energy value released during combustion (suitability)
- – Galling and Friction Ignition, development of frictional resistance (moving parts)
- – Mechanical Impact Ignition, ignition by collision of material particles
- – Electric Arc Ignition, ignition by an electric arc created by a short circuit
- – Flow Friction Ignition, flow-generated heat that triggers an ignition
- – Electrostatic Discharge Ignition by an electric arc caused by a static discharge
- – Sound energy discharge, rocket ignition systems
- – Storm
- – Secondary fire that spreads.
All these effects can cause inflammation. It is therefore recommended never to take the risk of inflammation lightly!
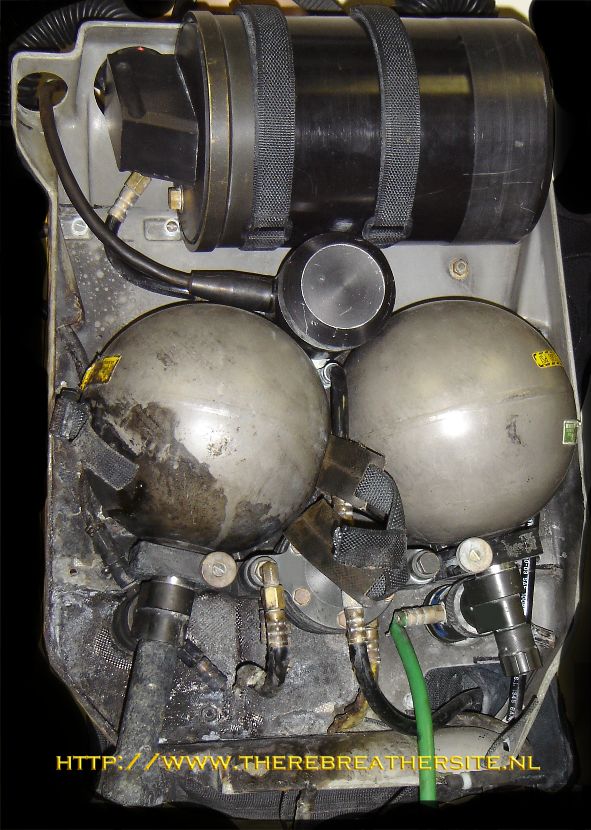
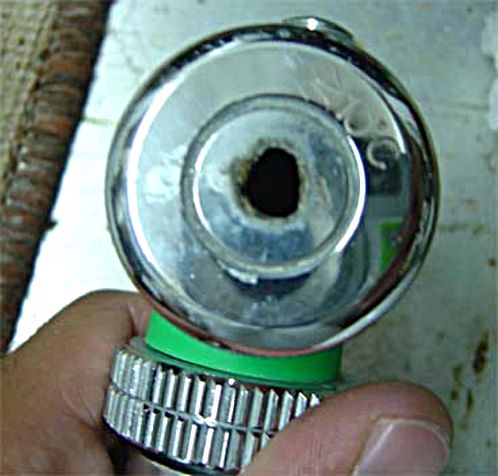
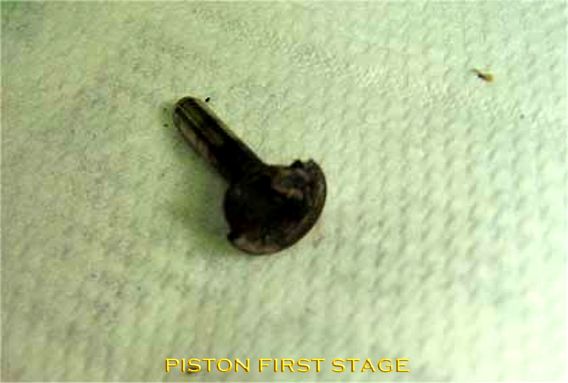
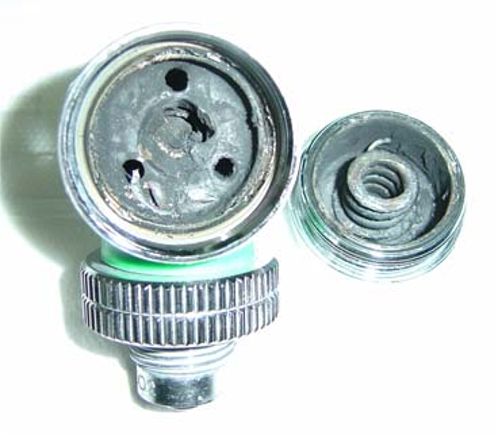
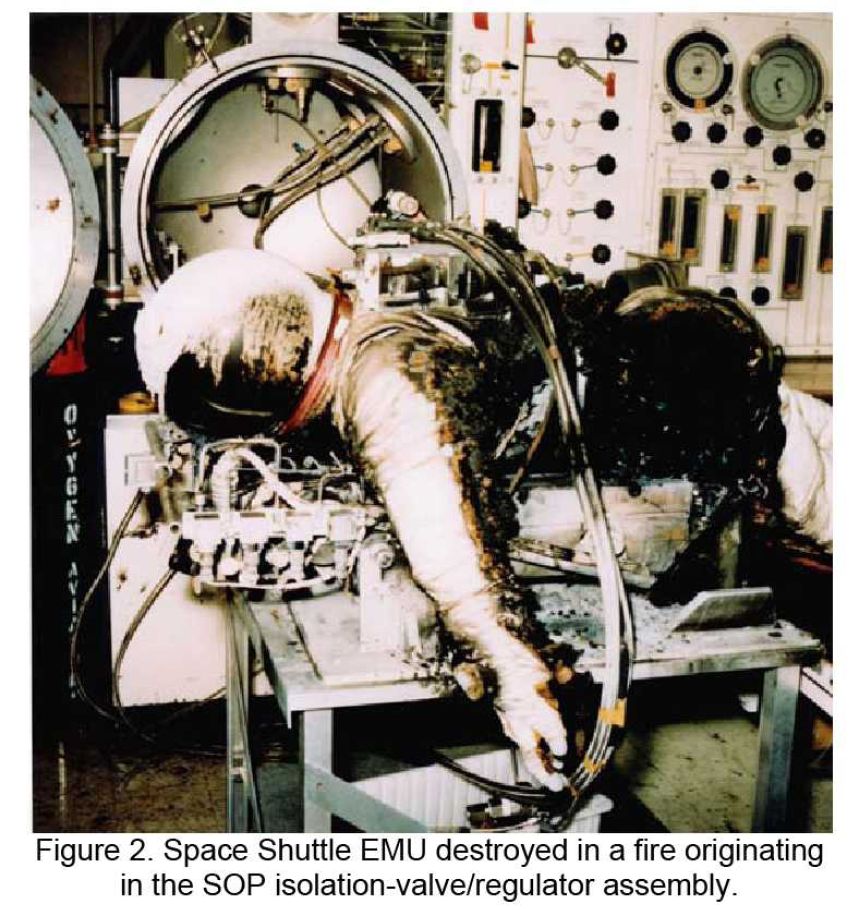
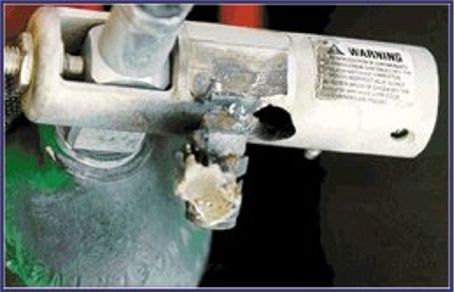
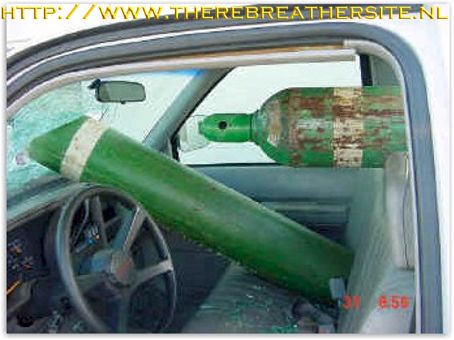
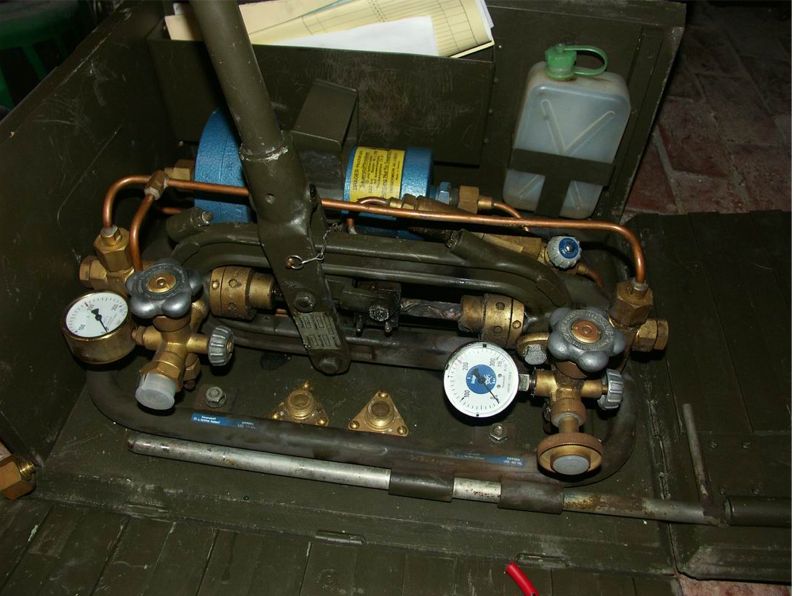
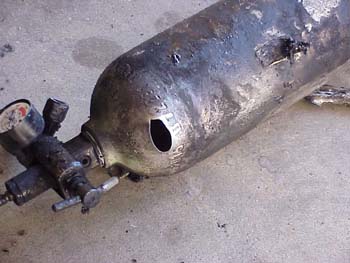
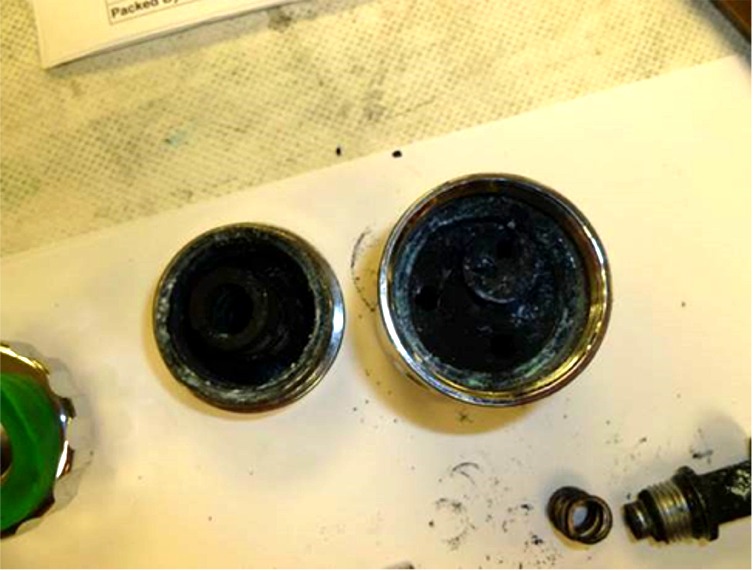
Relevant legislation, regulations and standards (Dutch/German):
- Arbobesluit artikel 3.5, Elektrotechnische, bedienings- en andere werkzaamheden aan of nabij een elektrische installatie voor de gezondheid schadelijke atmosferen.
- Arbobesluit artikel 3.6, Vluchtwegen en nooduitgangen.
- Arbobesluit artikel 3.7, Veilig gebruik vluchtwegen en nooduitgangen.
- Arbocatalogus Werken onder overdruk.
- Bouwbesluit.
- Besluit medische hulpmiddelen.
- Wet milieubeheer (WM).
- Wet algemene bepalingen omgevingsrecht (Wabo).
- ATEX 95, beschrijft voorschriften voor apparaten (elektrisch en niet-elektrisch) en beveiligingssystemen op plaatsen (“zones”) waar stof- of gasexplosiegevaar kan optreden.
- ATEX 137, beschrijft voor werkgevers de minimum veiligheidseisen om een gezonde en veilige werkomgeving te creëren voor werknemers die door explosieve atmosferen gevaar kunnen lopen.
- PGS 15, Opslag van verpakte gevaarlijke stoffen, richtlijn voor opslag en tijdelijke opslag met betrekking tot brandveiligheid, arbeidsveiligheid en milieuveiligheid.
- NPR 7910, Gevarenzone-indeling met betrekking tot explosiegevaar – Deel 1: Gasexplosiegevaar.
- NEN-EN-ISO 7396-1, Pijpleidingsystemen voor medische gassen – Deel 1: pijpleidingsystemen voor gecomprimeerde medische gassen en vacuüm.
- NEN-EN 50104: 2010, Electrical apparatus for the detection and measurement of oxygen – Performance requirements and test methods.
- NEN 3268:1984, Gasflessen voor industriële toepassing – Afsluiters, nippels en wartels – hoofdafmetingen en aansluitmaten.
- NEN 144-1:2000, Respiratory protective devices – Gas cylinder valves – Part 1: Thread connections for insert connector.
- NEN 144-3:2003, Respiratory protective devices – Gas cylinder valves – Part 3: Outlet connections for diving gases Nitrox and oxygen.
- DIN 477-1; Flaschenventil-anschlussformen.
ASTM (US) - ASTM G 63, Guide for Evaluating Nonmetallic Materials for Oxygen Service
- ASTM G 72, Test Method for Autogenous Ignition Temperature of Liquids and Solids in a High-Pressure Oxygen-Enriched Environment
- ASTM G 74, Test Method for Ignition Sensitivity of Materials to Gaseous Fluid Impact
- ASTM G 88, Guide for Designing Systems for Oxygen Service
- ASTM G 93, Practice for Cleaning Methods for Material and Equipment Used in Oxygen-Enriched Environments
- ASTM G 94, Guide for Evaluating Metals for Oxygen Service
- ASTM G 114, Practice for Aging Oxygen-Service Materials Prior to Flammability Testing
- ASTM G 120, Practice for Determination of Soluble Residual Contamination in Materials and Components by Soxhlet Extraction
- ASTM G 121, Practice for Preparation of Contaminated Test Coupons for the Evaluation of Cleaning Agents
- ASTM G 122, Test Method for Evaluating the Effectiveness of Cleaning Agents
- ASTM G 124, Test Method for Determining the Combustion Behavior of Metallic Materials in Oxygen-Enriched Atmospheres
- ASTM G 125, Test Method for Measuring Liquid and Solid Material Fire Limits in Gaseous Oxidants
- ASTM G 126, Terminology Relating to the Compatibility and Sensitivity of Materials in Oxygen-Enriched Environments
- ASTM G 127, Guide for Selection of Cleaning Agents for Oxygen Systems
- ASTM G 128, Guide for Control of Hazards and Risks in Oxygen Enriched Systems
- ASTM G 131, Practice for Cleaning of Materials and Components by Ultrasonic Techniques
- ASTM G 136, Practice for Determination of Soluble Residual Contaminants in Materials by Ultrasonic Extraction
- ASTM G 145, Guide for Studying Fire Incidents in Oxygen Systems
- ASTM G 175, Test Method for Evaluating the Ignition Sensitivity and Fault Tolerance of Oxygen Regulators Used for Medical and Emergency Applications
- ASTM D 2963, Test Method for Measuring the Minimum Oxygen Concentration to Support Candle-like Combustion of Plastics (Oxygen Index)
- ASTM D 4809, Test Method for Heat of Combustion of Liquid Hydrocarbon Fuels by Bomb Calorimeter (Precision Method).
EIGA (Europe)
- Doc. 4/00, Fire Hazards of Oxygen and Oxygen Enriched Atmospheres
- Doc. 10/81, Reciprocating Compressors for Oxygen Service. Code of Practice
- Doc. 13/02, Oxygen Pipeline Systems
- Doc. 27/01, Centrifugal Compressors for Oxygen Service. Code of Practice
- Doc. 33/06, Cleaning of Equipment for Oxygen Service: Guideline
- Doc. 51/02, Management of Change
- Doc. 89/06, Safe Use of Medical Oxygen Systems for Supply to Patients with Respiratory Disease
- Doc. 104/03, Safe Principles for Pressure Regulators for Medical Oxygen Cylinders
- Doc. 128/04, Design and Operation of Vehicles Used in Medical Oxygen Homecare Deliveries
- PP-14, Definitions of Oxygen Enrichment/Deficiency Safety Criteria
- Info 15/08E, Safety Principles of High Pressure Oxygen Systems
- Info 16/00, Fire in Regulators for Oxygen in Industrial Service
- NL 71/99, Oxygen for Healthcare/CO2 Cylinders with Quick-Opening Valves
- NL 72/00, Filters in Oxygen System/Excessive Pressure—Small Tanks
- NL 73/00, Oxygen Enrichment in Water/Silane Cylinder Safety
- NL 79/04/E, Hazards of Oxygen Enriched Atmospheres/EIGA Campaign Highlighting the Hazards of Oxygen Enriched Atmospheres
- PR 01/03, Oxygen Deficiency Presentation.

Therebreathersite was founded by Jan Willem Bech in 1999. After a diving career of many years, he decided to start technical diving in 1999. He immediately noticed that at that time there was almost no website that contained the history of closed breathing systems. The start for the website led to a huge collection that offered about 1,300 pages of information until 2019. In 2019, a fresh start was made with the website now freely available online for everyone. Therebreathersite is a source of information for divers, researchers, technicians and students. I hope you enjoy browsing the content!