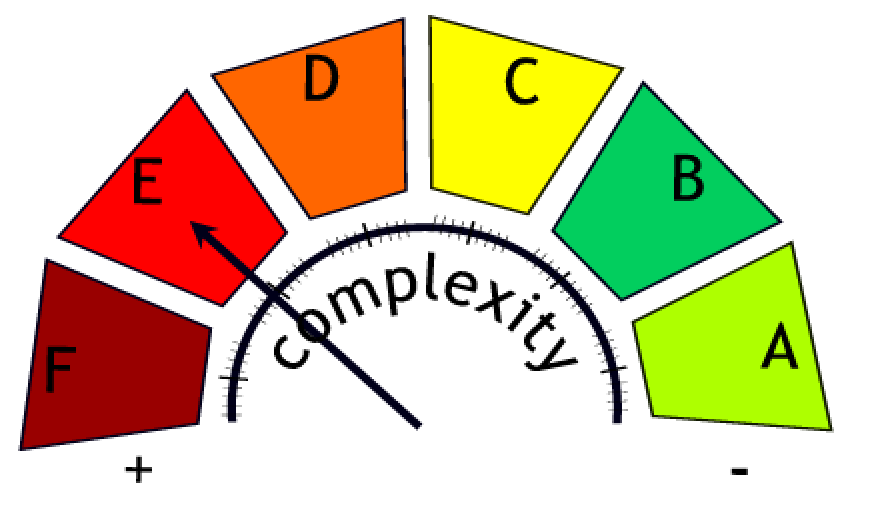
The name Naszogen was chosen by the company INHABAD GmbH from Berlin around 1930. Inhabad introduced a process that did not use gaseous oxygen in cylinders, but a chemical oxygen generating preparation. This process and the line of associated devices were called Naszogen devices.
Inhabad GMBH compared the technology with existing devices that used pressurised oxygen carried in cylinders on the device. The briquettes used by Inhabad would produce an amount equivalent to a pressure of 350 bar in the same cylinder compared to the 150 bar systems used at the time. Inhabad used this as a commercial selling point. The advertisement used with the Naszogen process was therefore called O2-350.
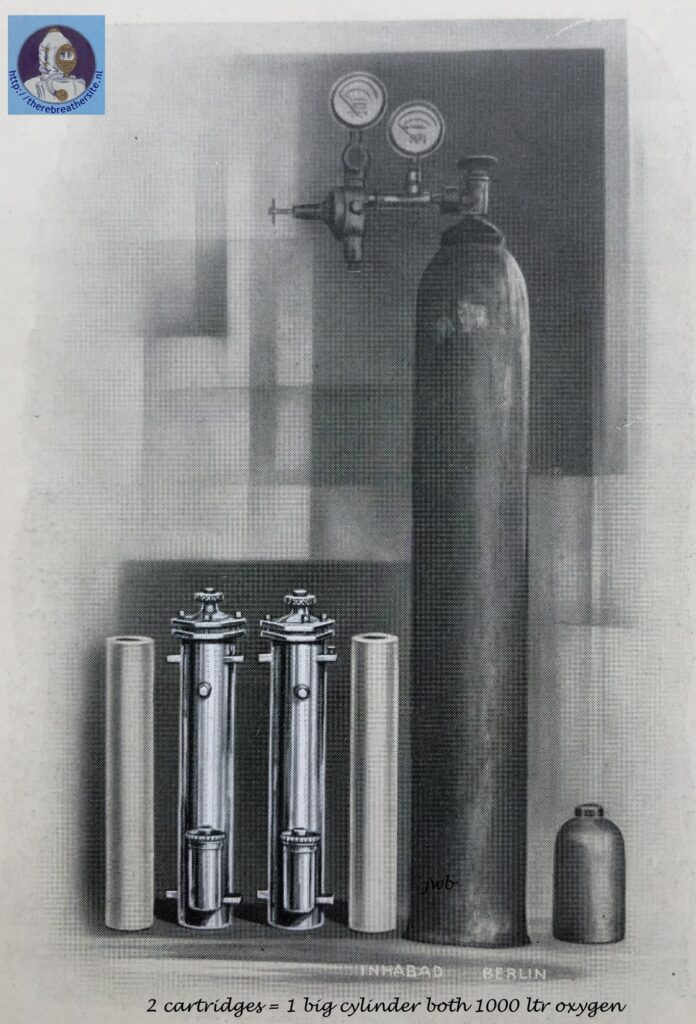
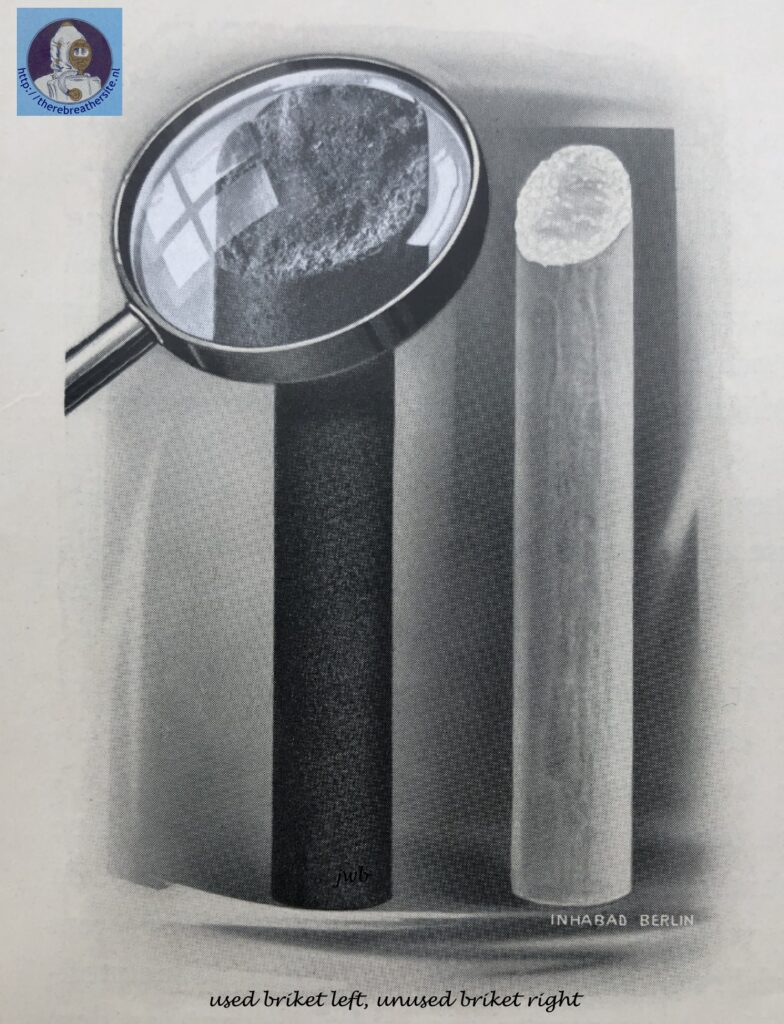
The article Superoxide in the vademecum of this website discusses the functioning of Superoxide. Superoxide is a chemical substance that produces oxygen under the action of water vapour and carbon dioxide. Initially, this seemed to be the ideal product to reduce the high costs of oxygen generation, compression and boosting. The efforts proved to be in vain, as devices such as the Pneumatophor and Drägerogen proved impractical. As described earlier on the page about Superoxide, it takes too much time to start the chemical process to produce oxygen. Also, the temperature and dust production caused by the process proved impractical for this application.
The chemical process to generate oxygen was based on the following equation:
KCLO3 = KCL + 3/2 O2
Potassium chlorate is a substance used to make explosives and therefore needs to be reduced in reaction speed. Inhabad mixed components with the product with the aim of slowing down the heat development, the melting and evaporation and conversion to KCL. This resulted in a method to generate oxygen for up to two hours with such a chemical preparation. The product was mixed and pressed into bars that were placed in a so-called developer. The process was started by an igniter, after which the oxygen production started almost immediately.
Dr. Ing. A. Hloch
In contrast to this type of oxygen-generating apparatus, a new, recently introduced device generates oxygen by thermal decomposition of alkali. chlorates. Here, the oxygen-forming process is independent of the breathing· activity, in contrast to alkali peroxide-type devices. in which this process is supposed to be regulated by the breathing rate. In the new device, as in equipment using compressed oxygen, carbon dioxide must be removed from the exhaled air by passing this air through cartridges (scrubber) containing granular caustic alkali. The new device is thus not based on the principle of simultaneous oxygen formation and carbon dioxide absorption in a single reaction. This indicates that, even after 25 years of development, it was not possible to apply this principle to gas protective devices .
A requirement for oxygen generation, in .gas protective devices , by thermal decomposition of chlorates according to the equation
KCLO3 = KCL + 3/2 O2
is that it must be controllable. A sudden, non-regulated evolution of the total amount of oxygen required for a certain use period, as previously proposed for gas protective devices, would make it necessary to store the oxygen. This could be achieved
a) under conditions of practically no pressure, in gas-tight containers, e. g. , rubber bags, which. however, cannot be used in portable equip-ment, because of their excessive volume (a 300 l capacity would be required for a 2-hour use period) or
b) under pressure, in thick-walled, heavy containers, requiring pressure-reducing valves for removal of oxygen. Both these situations are to be avoided, however.
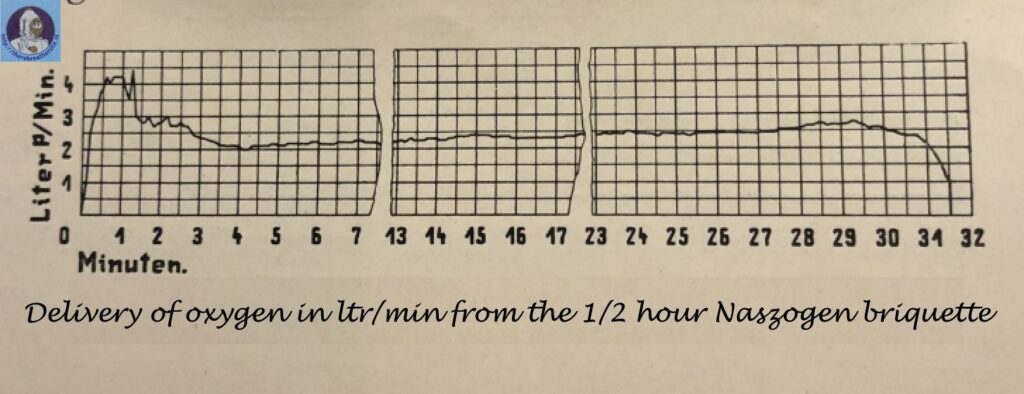
The oxygen supply must, therefore, be regulated so that the oxygen requirements of the wearer are satisfied both during light and heavy work conditions. According to specification guidelines for gas-protective devices used in mines, issued by the Prussian Commission for Mine Safety, such devices should be designed for an average oxygen consumption of 1. 3 l/min, corresponding to a marching rate of 6 km/hr. In addition, they should provide enough oxygen for a peak consumption of 3 l/min for a minimum of 1 min and a maximum of 5 min. In the new device:, oxygen generation from chlorate occurs at a rate of 2. 5 l/min, which satisfies total oxygen requirements for medium and high work conditions. During peak activity, corresponding to an oxygen demand of 3 l/min, the wearer obtains additional oxygen from a breathing bag which is inserted into the breathing cycle, and has a capacity of 7 liters. This bag is filled with oxygen produced in increased amount during the starting period. Thus, during the period of peak activity lasting at the most 5 min, 2,5 l of oxygen is removed from the bag, with about 1 liters still remaining.
A constant flow of oxygen, at a rate of 2. 5 l/min, is obtained by restricting decomposition to a corresponding amount of chlorate per minute. This is achieved by use of a chlorate or perchlorate rod. The reaction is started at one end of the rod by an initial supply of heat. The heat liberated during decomposition according to equation [1] (this heat can be increased by the heat of oxidation from oxidizable additives, e. g. , metals) then causes the decomposition of adjacent layers. In this manner, the reaction propagates along the entire length of the rod. However, the quantities of heat formed during the progressing exo-thermic reaction are additive and this causes a gradual increase in the rate of decomposition. As a result, constant oxygen evolution is impossible and, under certain conditions, the process may even become explosive. This possibility is eliminated by addition of substances which, while undergoing the exothermic main reaction, also undergo an endothermic change brought about by this exo-thermic reaction such as melting, vaporization, decomposition or endothermic reaction with other compounds. In this manner, it is possible to produce a regulated oxygen stream which is sufficiently constant for the purpose considered here (Fig. 1). It is even possible to increase or decrease the flow rate at will; for example, during the first 2-3 minutes after oxygen evolution is started by percussion, the oxygen rate may be raised up to 6 l/min to fill the breathing bag and to flush the apparatus with oxygen. Because of this, no “breathing induction” period is required as is the case with peroxide-based devices, the apparatus being immediately ready for use which is of paramount importance in nearly all cases.
To ensure propagation of the reaction, it is necessary to maintain a constant chlorate decomposition temperature (this temperature is reduced to about 300° C by use of catalysts). This is accomplished by suitable selection of the exotherm- and endoththerm-controlling additives and by proper arrangement of the insulating jackets. Tests at low temperatures (-42 ° C) have shown that cooling does not interrupt oxygen evolution; by contrast, the peroxide devices fail at temperatures slightly below 0°C. For this reason, the “Naszogen” process may be used also· to supply oxygen to underwater diving equipment and high-altitude breathing devices for fliers.
The operation of such a gas-protective device, designed for a 1-hour use period, is illustrated in the figure below.
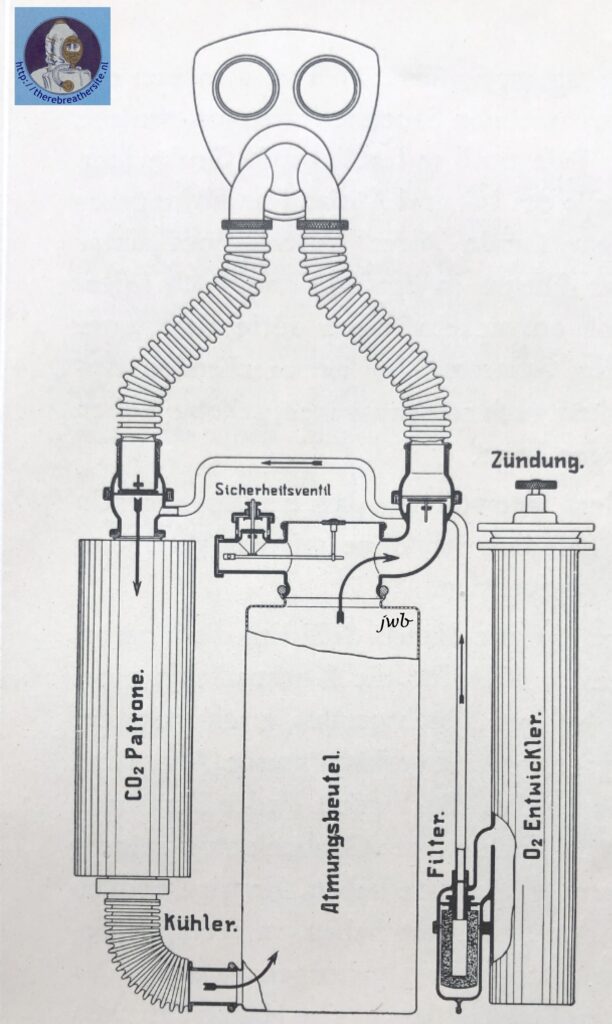
The exhaled air passes through the right-hand corrugated tube and an exhaling valve into the carbon dioxide-adsorbing cartridge. · The oxygen pronounced continuously in the “02 generator” at a rate of 2. 5 l/min, passes through a ceramic candle filter where entrained dust particles (primarily KCl) are retained and enters the breathing cycle downstream from the exhaling valve; here it comes together with the exhaled air, and the resulting mixture then passes through the carbon dioxide absorbing cartridge where contaminants, mainly consisting of O2 – 0,5% CO2 , are removed. The regenerated air leaving the cartridge enters a breathing bag of approximately 7 l capacity which serves as a reservoir for the breathing air and for a certain amount of reserve oxygen for use during periods ,of peak activity. The air then passes through the inhaling valve and the left-hand corrugated tube and is inhaled by the user. Unused oxygen escapes through an automatic membrane-type safety valve actuated by a lever.
It is important that the temperature of the inhaled air be low. Absorption of contaminants from the ex -haled air in the alkali cartridge generates about 126 cal at a marching rate of 7 km/hr; as a result, the air leaving the cartridge is heated as high as 120° C. Before this air reaches the user’s mouth, this temperature must be reduced to below 50° C. In the case of “chemical” , self-contained devices, this heat is augmented by that produced during oxygen generation. Devices using sodium peroxide generate for example under the above-cited work conditions, more than 400 calories; as a result the breathing air is heated to such a high temperature that attempts to cool this air during its short travel to the mouth under fast breathing conditions have thus far encountered insuperable difficulties.
The heat given off during oxygen generation from chlorates amounts to only about 130 cal/hr and exerts only a minor effect on the breathing air. As regards the location of the heat sources, devices of this type differ substantially from those just described in that, in the peroxide devices, these sources are located in the breathing cycle itself, whereas in the chlorate devices they are outside this cycle. In other words, the inhaled air is heated only by the heat introduced with the oxygen (2.5 l/min). This heat amounts to only about 2 – 3 calories and is almost negligible compared to that given off during absorption of the contaminants from the exhaled air.
The principle considered ideal for self-contained equipment has not been adopted in this new device. This principle is based on the use of the carbondioxide and moisture content of the exhaled air as a measure of oxygen demand, and as a means for controlling oxygen generation correspondingly. Neglecting the technical difficulties which preclude realization of such devices, the problem is incorrectly formulated from a physiological standpoint. Thus, for example, the increased amounts of carbon dioxide and water formed as a result of increased work produce an increased quantity of oxygen only after this quantity was needed by the body. In the continuing development of peroxide devices, this has been taken into account by partially hydrating the sodium peroxide. In this manner, oxygen evolution occurs also under the effect of heat and not only in dependence of the amount of carbon dioxide and water in the air.
References:
- M. Bamberger and F. Bock, Z. Angew. Chem. Vol. 17, p. 1426, 1904.
2. G. Stampe and E. Horn, Z. Angew. Chem. Vol. 42, p. 776, 1929
The next article describes the Inhabad proces in later period with the obtained patents and with positive and negative aspects of the proces
Oxygen evolution through processes independent of exhalation.
The Naszogen device developed by Inhabad GMBH Berlin, in 1929, is intended to eliminate the aforementioned fundamental deficiencies of the superoxide devices. The decomposition of a specially composed, briquette-like chlorate-perchlorate body serves to produce oxygen (German Patent. a. J. 38341 Kl. 12 i 14 and English Patent. 341975 [1929]). By adding catalysts, e.g. cobalt oxide, and reducing metal powders, the decomposition temperature of the chlorate is reduced to 300°. After local heating by impact initiation, the reaction propagates in the rod-shaped body with a constant oxygen evolution of 2.5 l/min. A slightly stronger delivery in the beginning helps over the start-breathing period. For peak physical performance, the contents of the spacious breathing bag (5-7l) provide sufficient reserves thanks to ample continuous dosing. The mode of operation of the device can be readily seen in the figure above. See also the article of Dr. A. Hloch, Ztschr. angew. Chem. 43, 732 [1930]. In view of the short time they have been in use, little is known about the practical use of the wet drawing apparatus, which has recently been produced in various forms. Certainly, this is a remarkable idea for respiratory protection. The advantage of the chlorate cartridge is its convenient storage, durability and automatic decomposition once initiated. On the other hand, once the briquette has been set in motion, it smoulders to an end without any possibility of supply adaptation when in need of lower demand or an interruption of breathing in the apparatus. The area of application is therefore to be sought especially where the use of the equipment is expected to last for a certain period of time (e.g. during high-altitude flights, long-lasting rescue work, etc.) and where value is placed on the light weight of the oxygen producing cartridge. A half-hour briquette (80/2) weighs 500 grams, a one-hour briquette (150/2) 800 grams, a two-hour briquette (320/2) 1.6 kg. The imminent exhaustion of the briquette is indicated by a stimulus signal (camphor smell). The briquettes are bullet-proof, which is important for military service. The development of chlorate briquettes is not new (cf. D.R.P. 184268 [1904], 223246 [1906], 194327 [1906], 243 367 [1909], 247093 [1909], 259 575 [1912], 244 839 [1910]); however, the arrangement of the wet-drawn device seems to be the first successful way of obtaining oxygen sufficiently free of chlorine and chlorine oxides for respiration purposes.
Added by JW Bech;
Around 1950, it became known that asbestos, when inhaled, has serious consequences for human health (mesothelioma). The patents obtained by Inhabad show that the mixture could function in the manner described above by mixing it with asbestos fibres (see attached patents). The heat jacket that formed the insulation between the actual cartridge and the outer jacket was also made of asbestos. Possibly for this reason, the Naszogen process never became a commercial success.
The next pages shows you the devices made in the Naszogen series and the patents obtained bij Inhabad for this products.
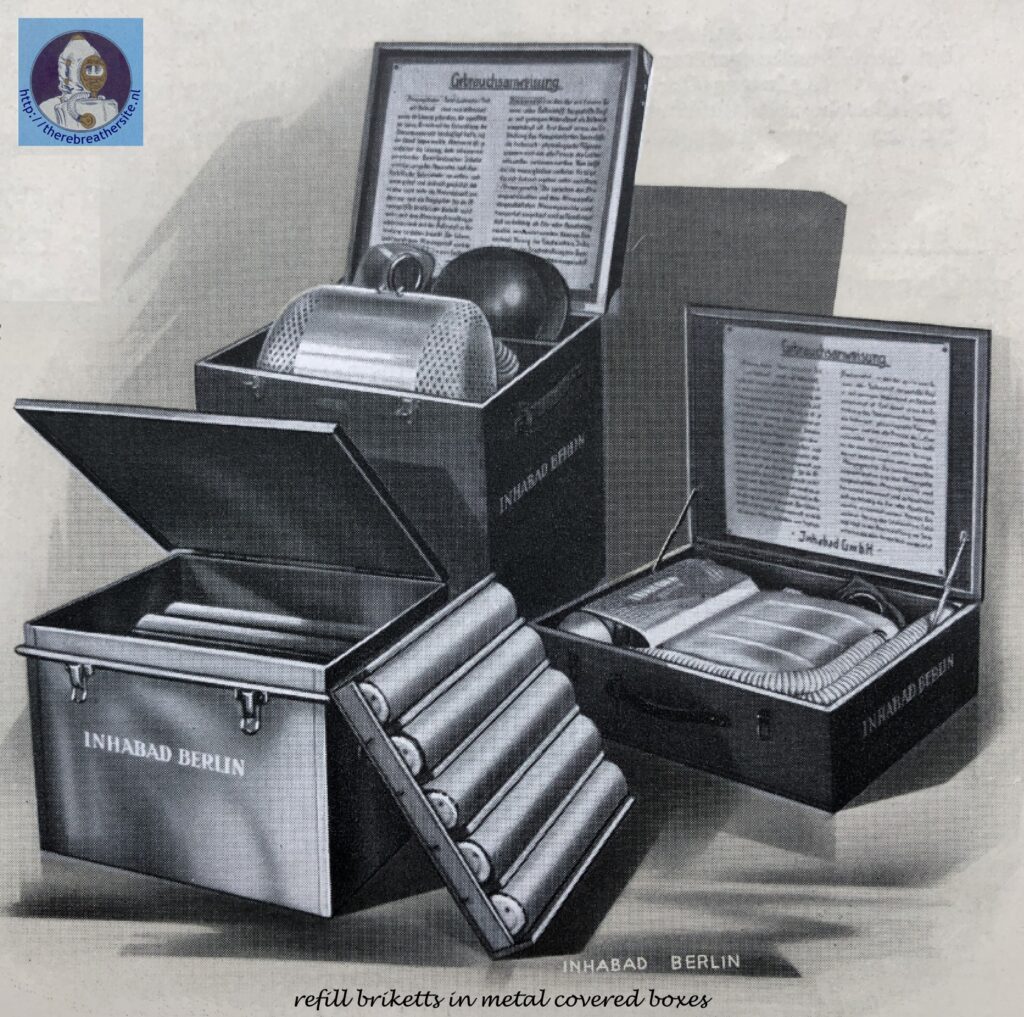
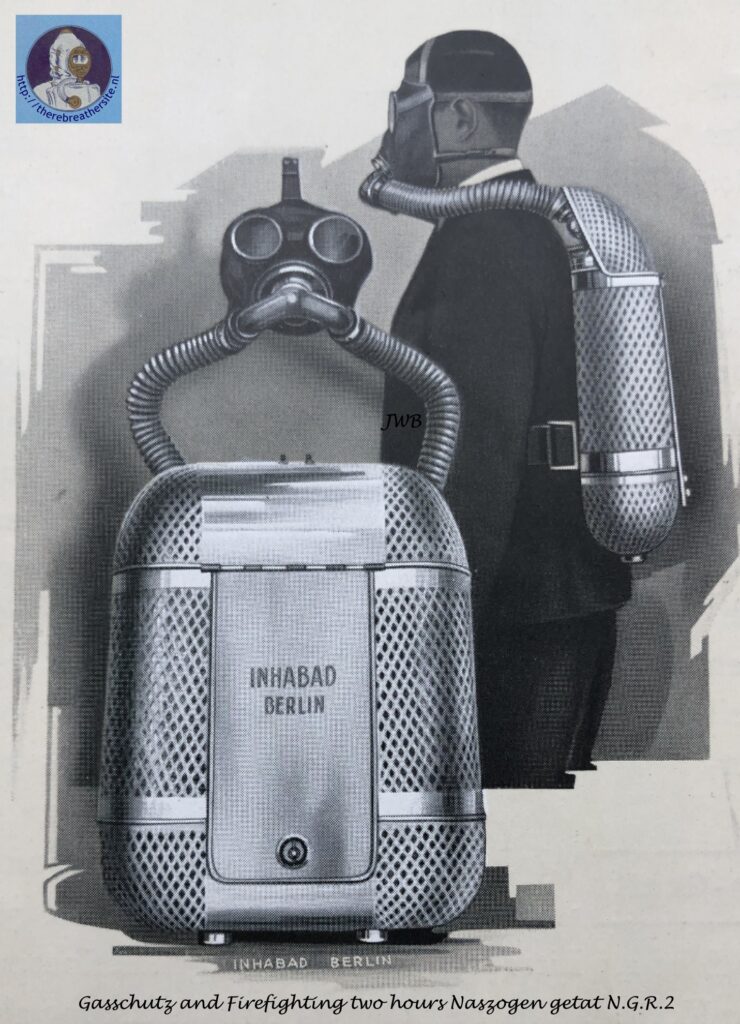
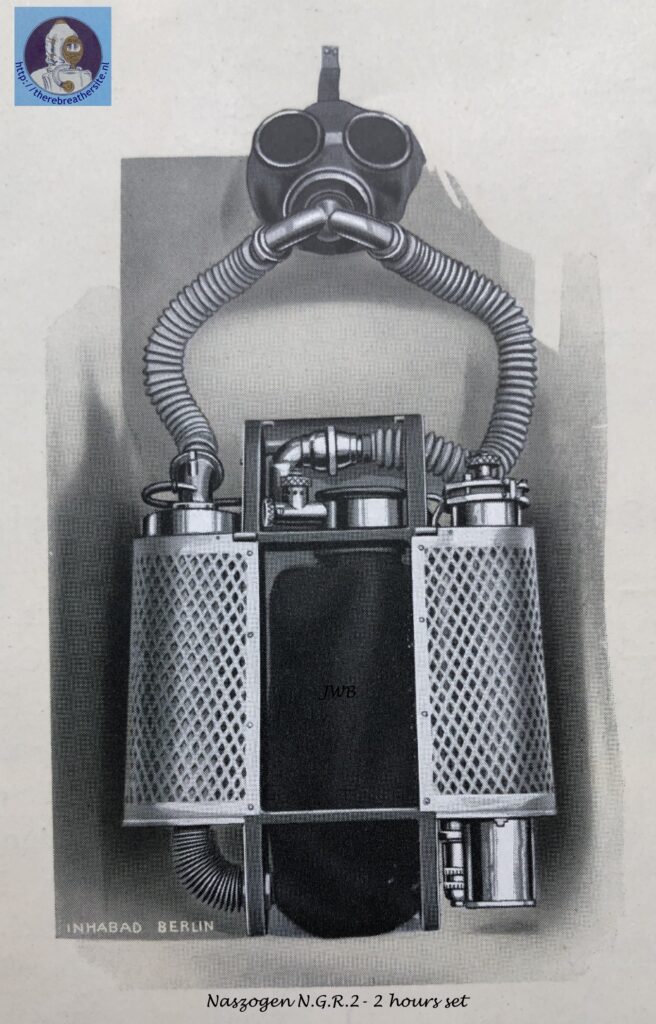
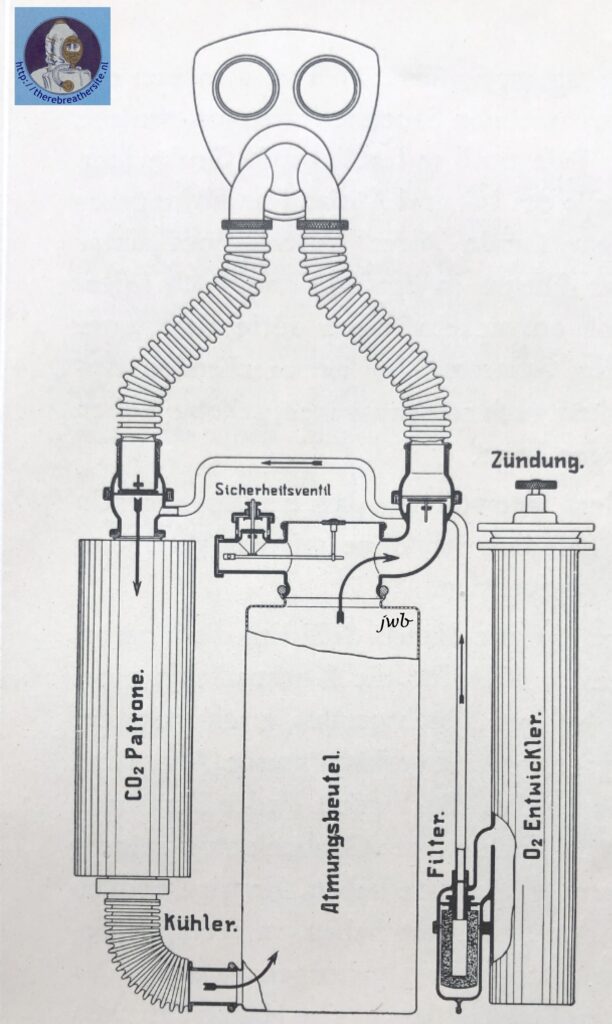
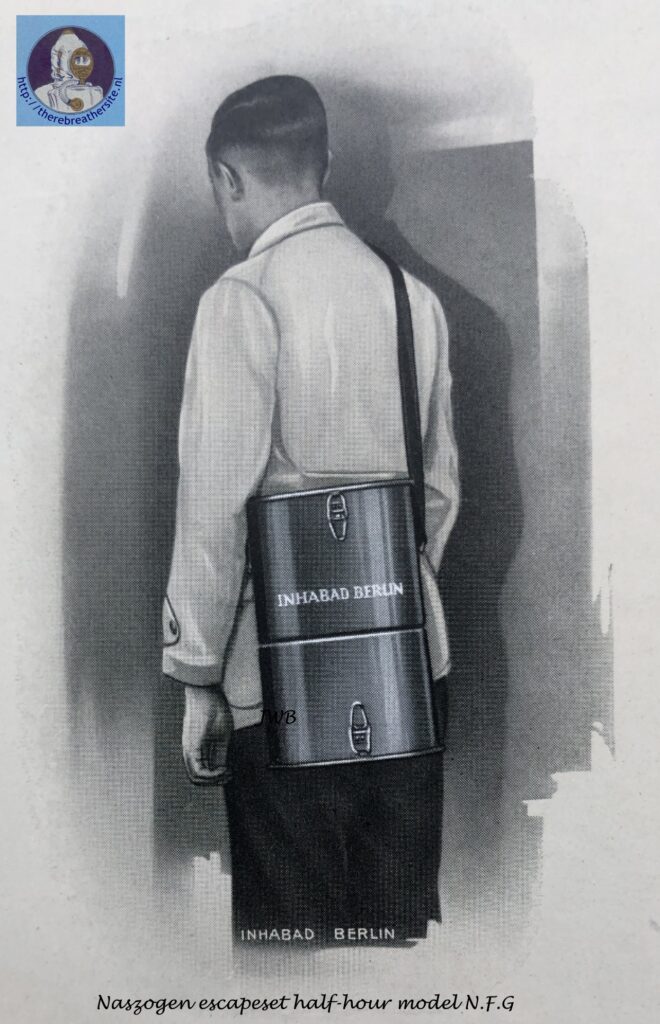
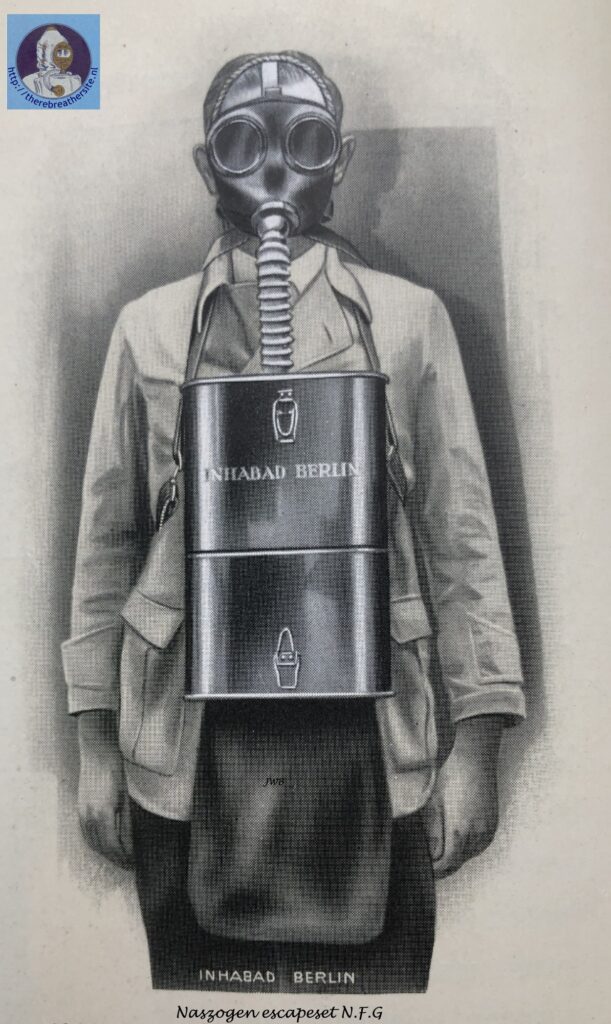
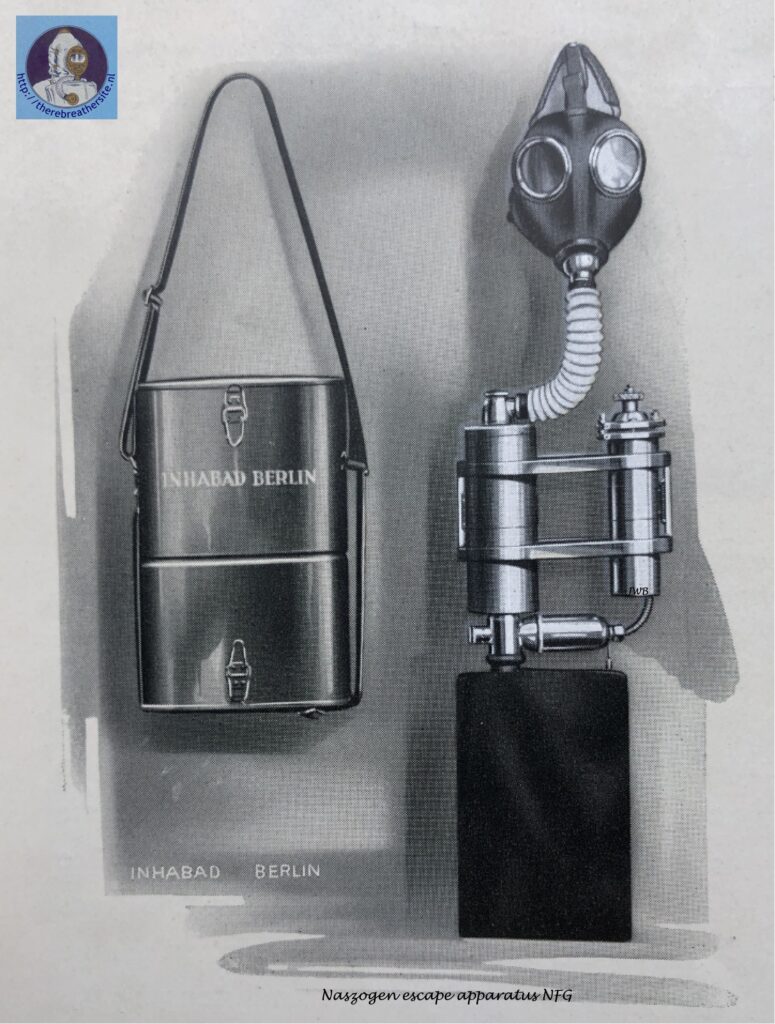
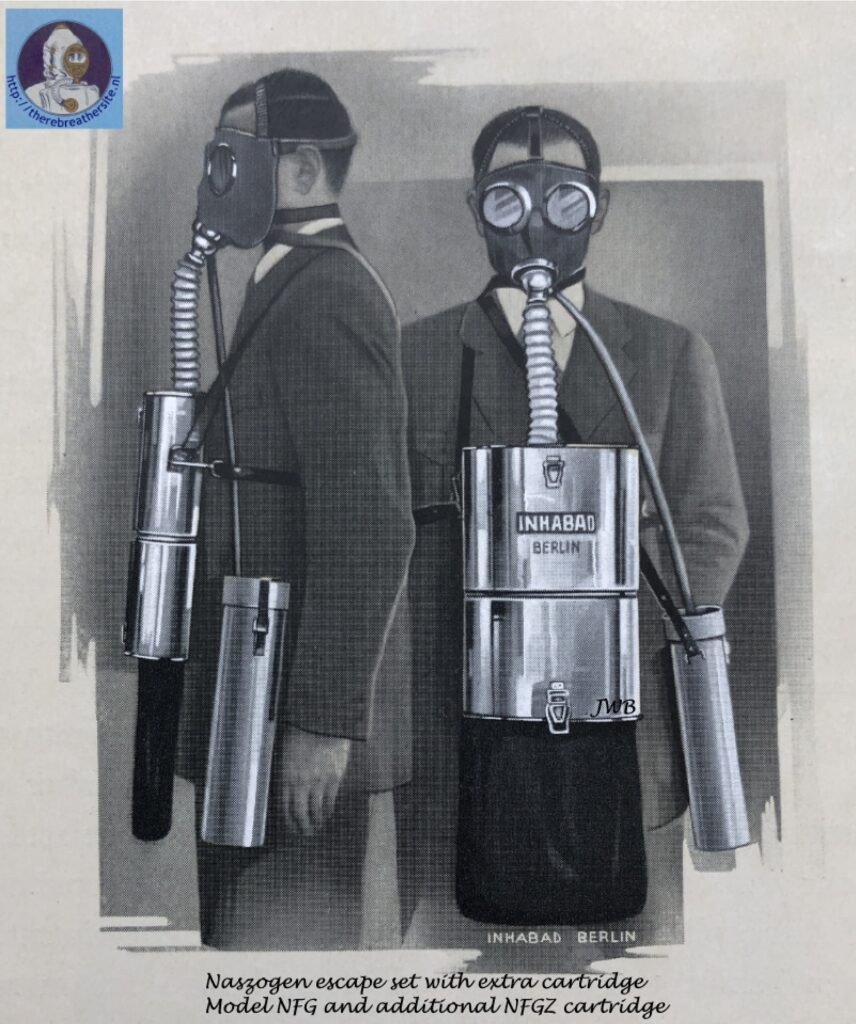
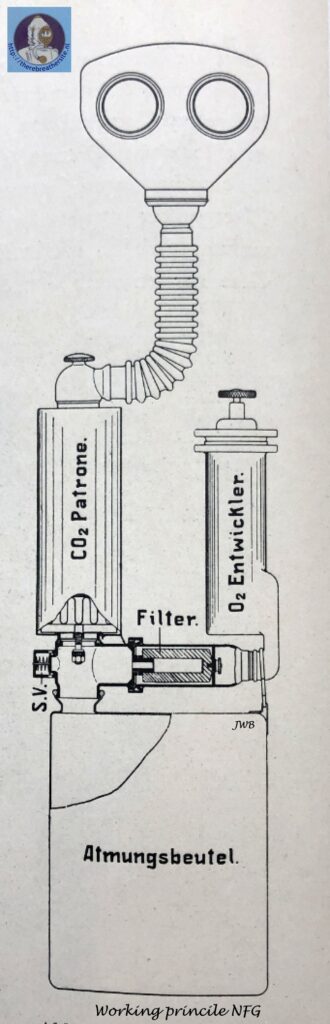
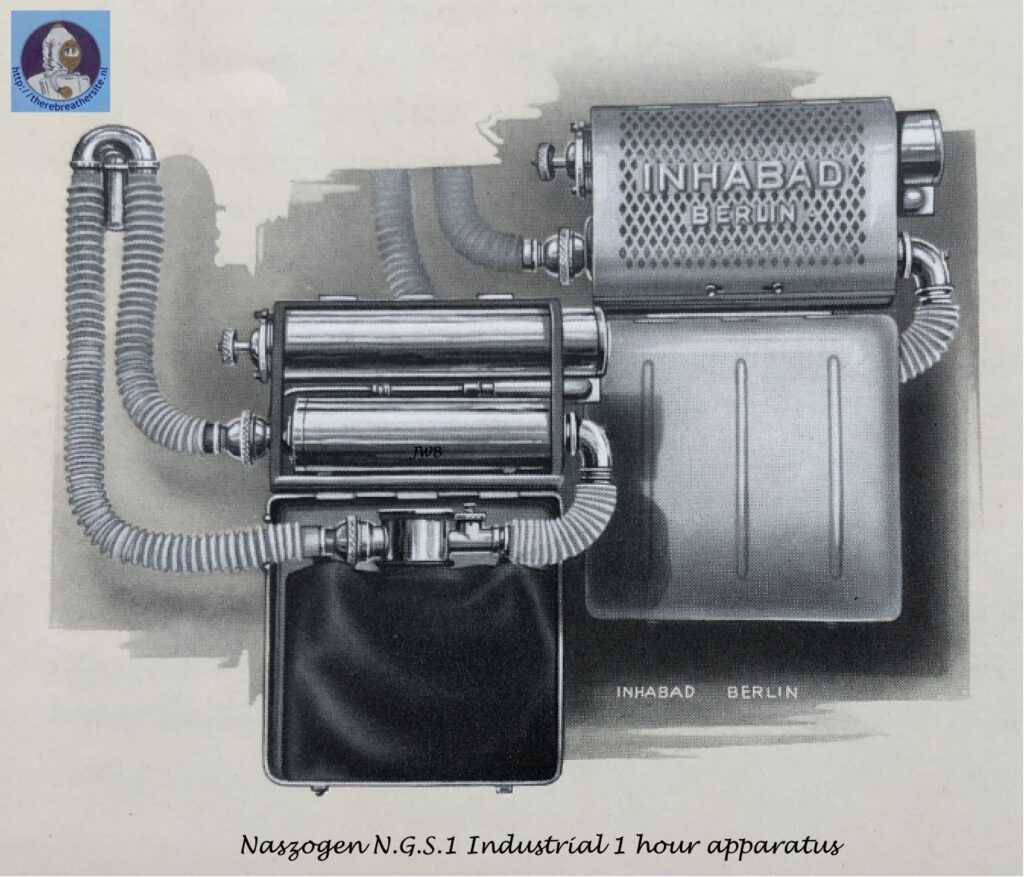
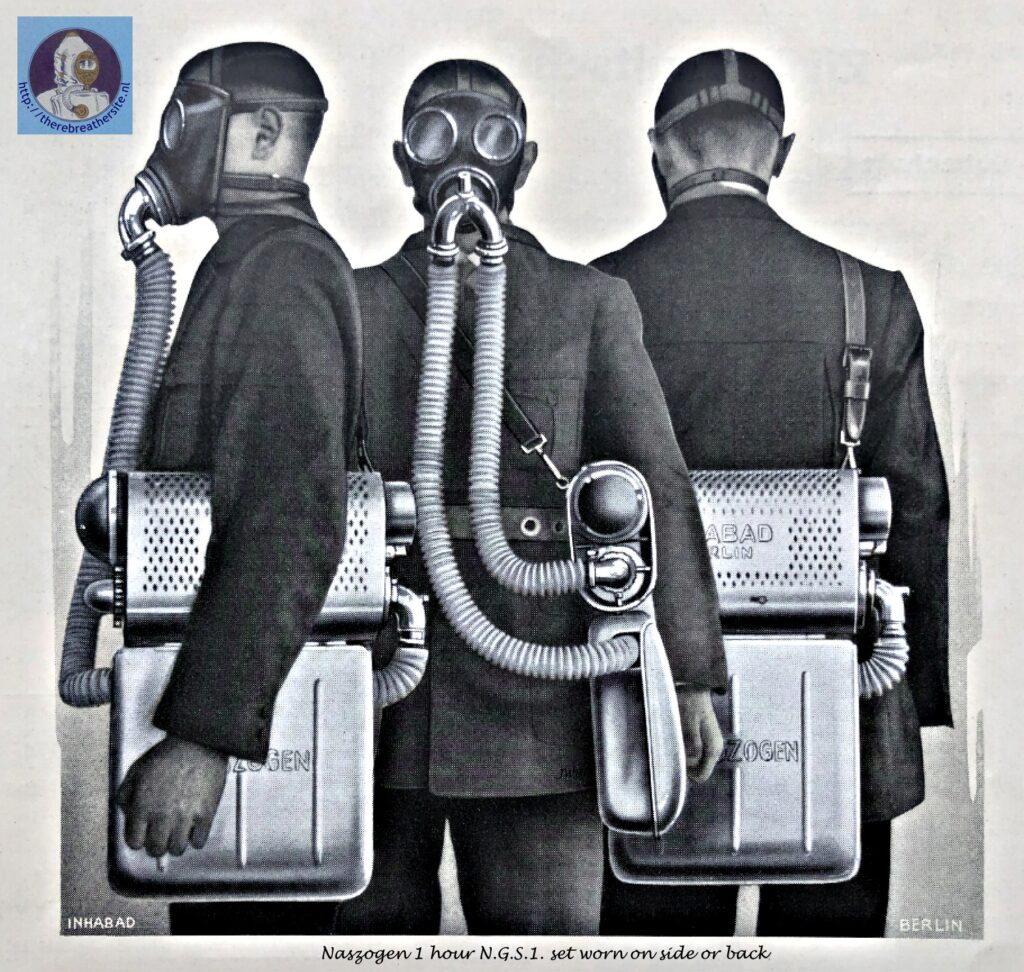
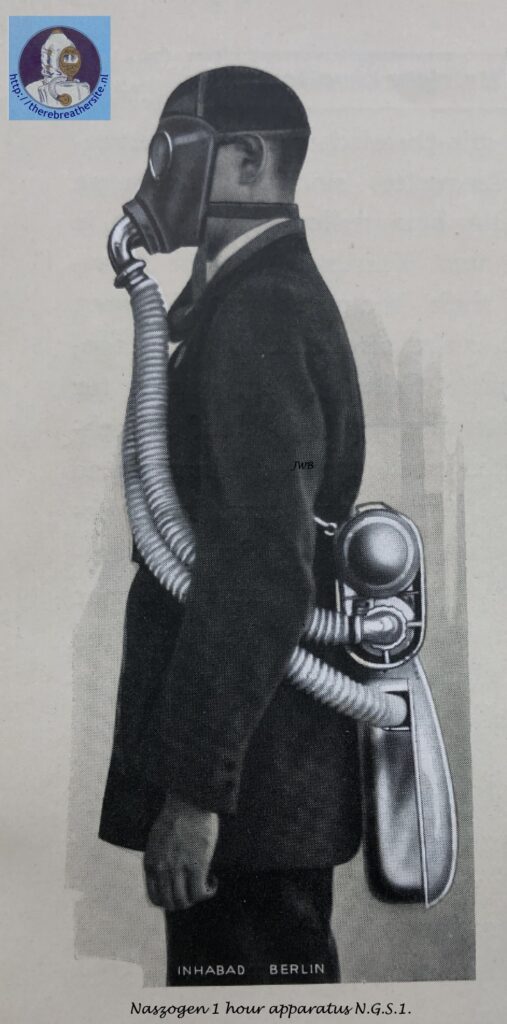
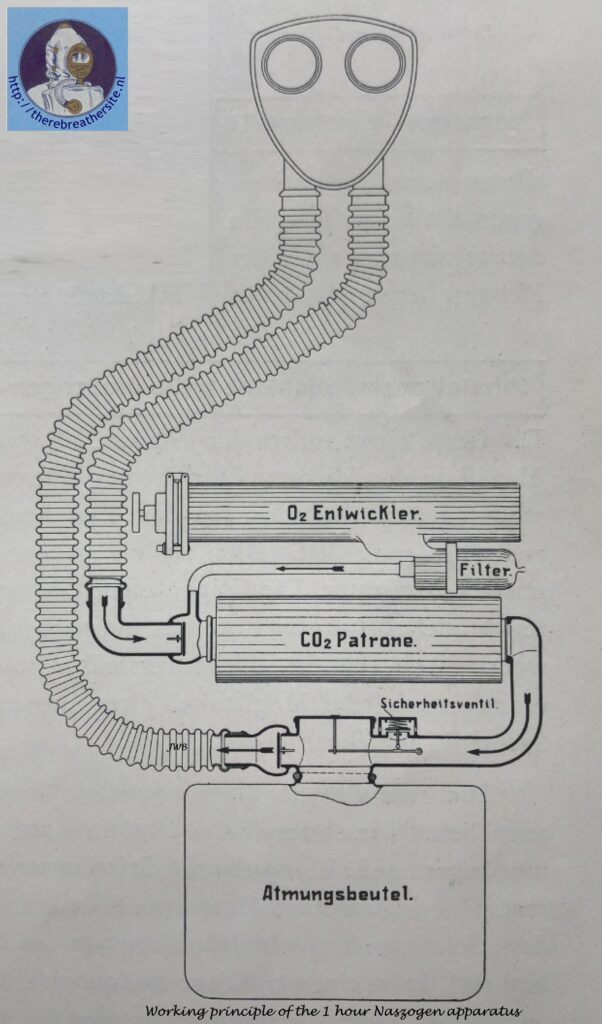
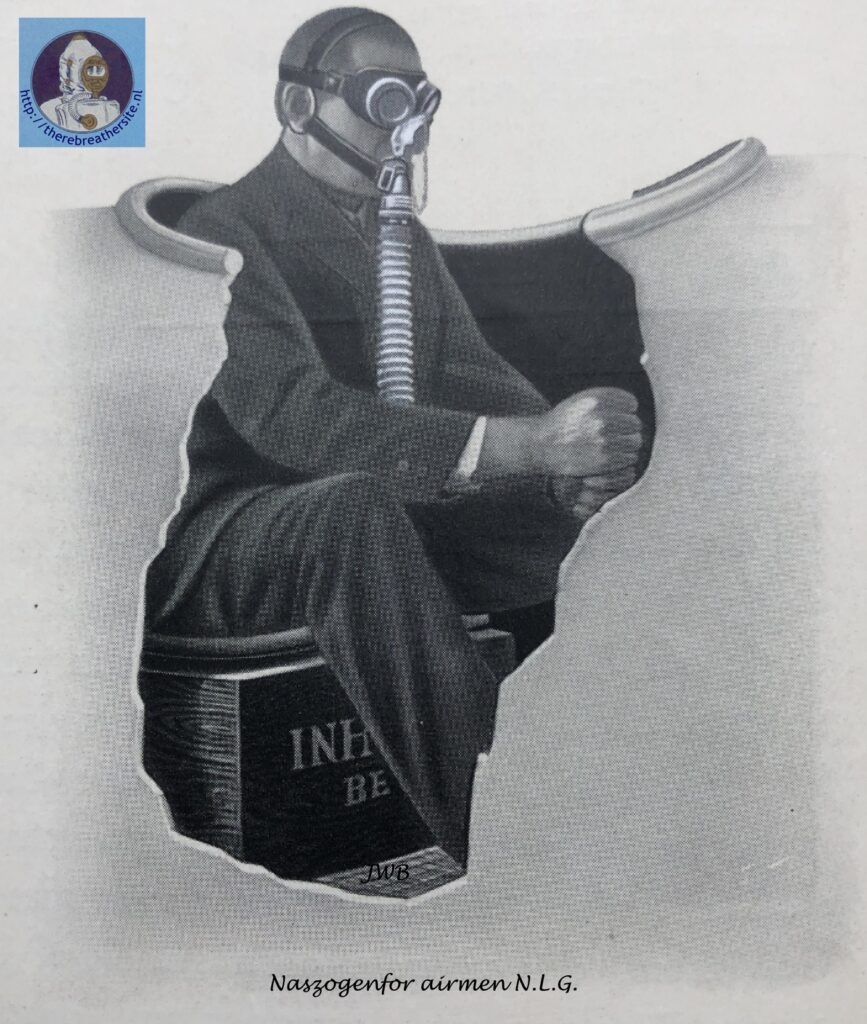
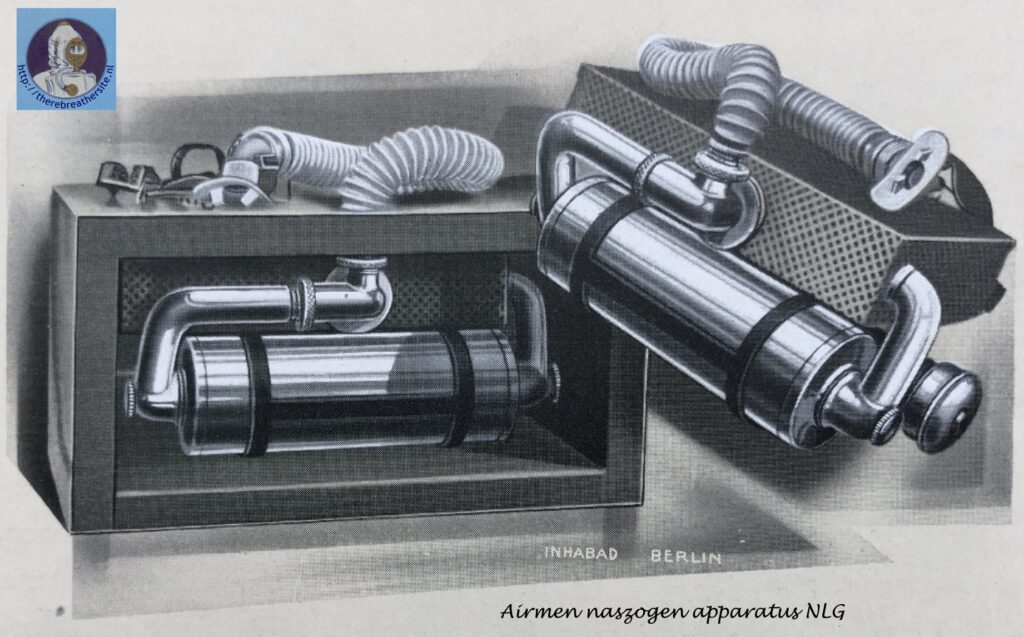
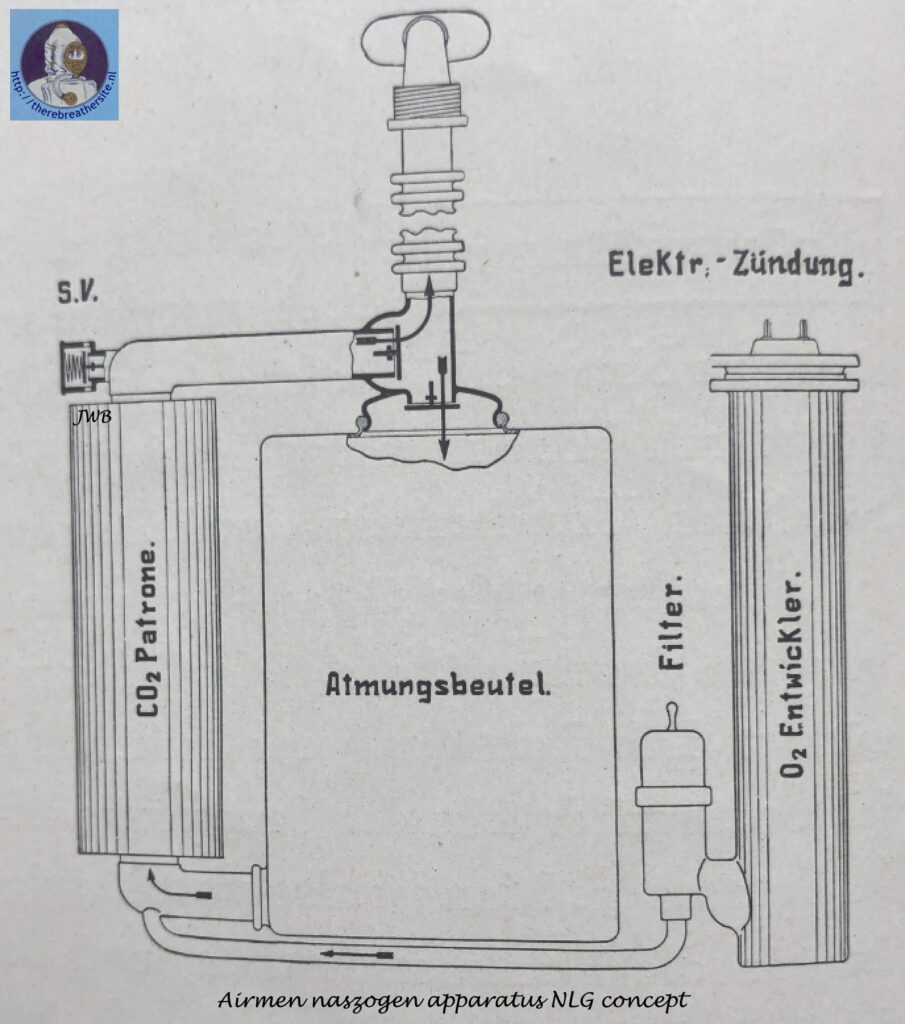
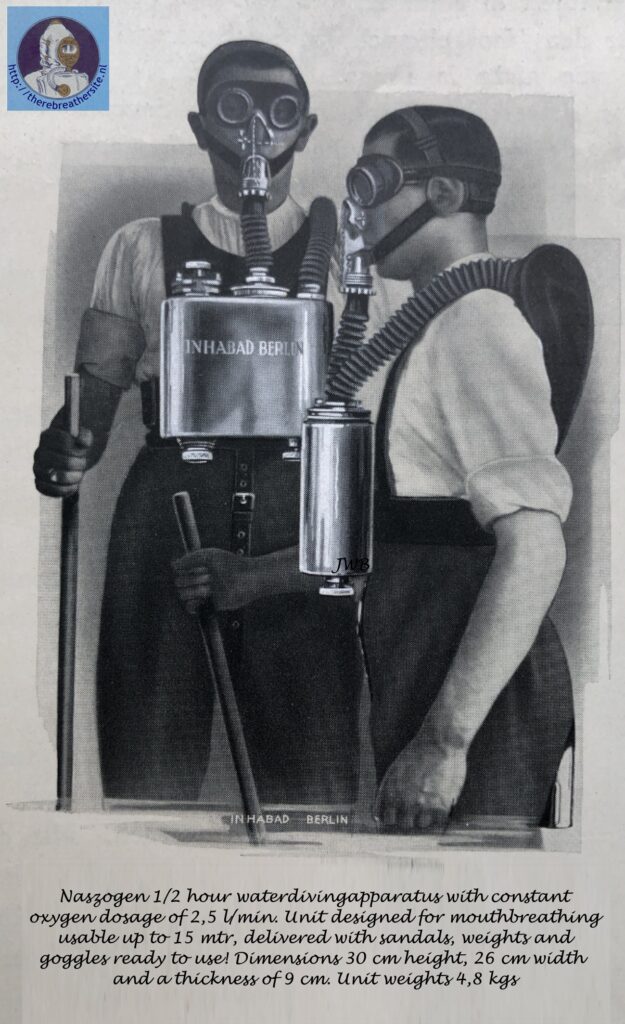
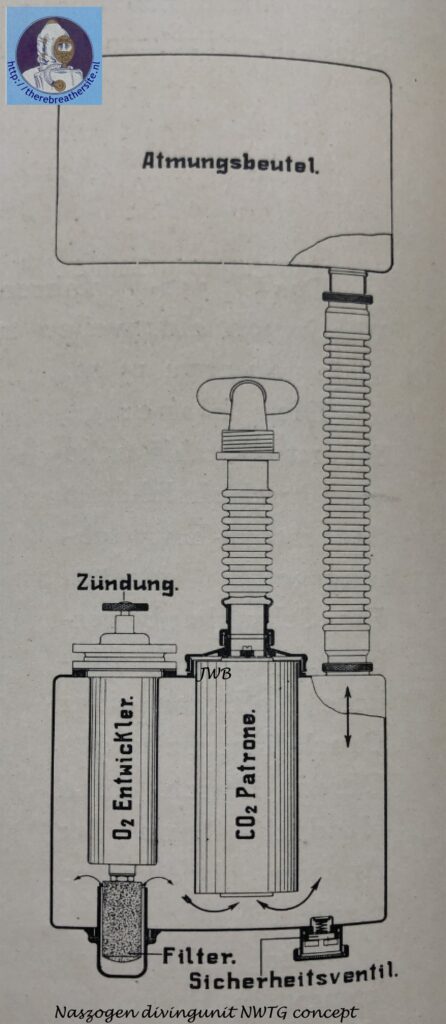

Therebreathersite was founded by Jan Willem Bech in 1999. After a diving career of many years, he decided to start technical diving in 1999. He immediately noticed that at that time there was almost no website that contained the history of closed breathing systems. The start for the website led to a huge collection that offered about 1,300 pages of information until 2019. In 2019, a fresh start was made with the website now freely available online for everyone. Therebreathersite is a source of information for divers, researchers, technicians and students. I hope you enjoy browsing the content!