Discovered by Daniël Rutherford and at the same time by Carl WIlhelm Scheele nitrogen is a colorless, odorless and tasteless gas.
Scheele made his discovery independently, but simultaneously with the English clergyman and scientist Joseph Priestley. However, Scheele is best remembered for his role in the discovery of oxygen, as described in his only book, Chemische Abhandlung von der Luft und dem Feuer (1777; “Chemical Treatise on Air and Fire”). Like most chemists, they were convinced that air consists of at least two different kinds of airs: one that sustains combustion and one that does not. Scheele measured the amount of the air suitable for combustion and found it to be about one-fourth of ordinary air. To isolate a pure sample of this gas, he tried heating different substances, such as mercury oxide, and black magnesia. He interpreted the experiments according to the prevailing phlogiston theory and called the gas “fire air.” The theory explained combustion by assuming the existence of a hypothetical substance, phlogiston, that left a material as it burned. Thus, when all of a material’s phlogiston had disappeared, combustion ceased.
About 79 percent of our atmosphere consists of nitrogen. The gas is artificially produced by air fission. Nitrogen has a 13-degree Celsius lower boiling point than oxygen, allowing it to be separated by fractionation.
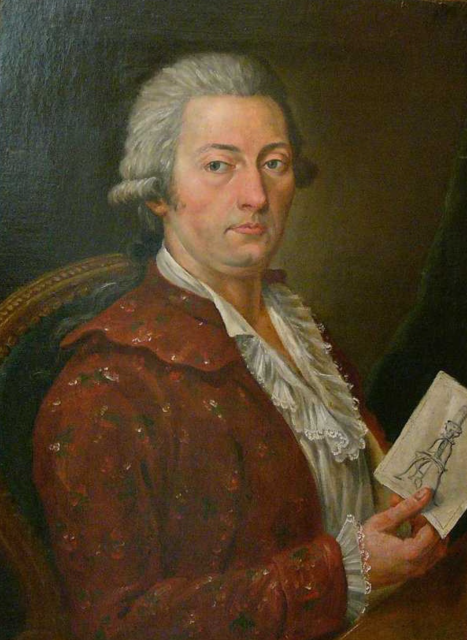
For diving and professional divers, nitrogen is the gas that is very decisive next to oxygen. Nitrogen is absorbed into the blood from the breathing gas. Nitrogen is absorbed into the blood and will be absorbed more and more into the blood due to the increasing pressure during a descent. The gas will dissolve into the blood under pressure. On ascent the ambient pressure will decrease and the gas will be released from the blood again. If we ascend too quickly, the gas can form bubbles that can cause blockages. This last phenomenon is known as decompression sickness. The theory of safe ascent focuses on preventing bubbles in the bloodstream. This is possible because the nitrogen can also leave the body via the lungs, provided that it can be discharged gradually. If we ascend too quickly, decompression sickness will occur.
Nitrogen can cause dizziness in addition to decompression problems. If the partial pressure of nitrogen rises above 3.6-4.0 bar, a diver will be caught in a nitrogen intoxication. A diver will suffer from this at a depth where there is a pressure of (3.6/0.79= 4.55 bar and 4.0/0.79 = 5.06) 35.5 and 40 meters. We divide the pressure at which the phenomenon of nitrogen intoxication occurs by partial pressure of nitrogen in breathing air. The depth is this pressure less the atmospheric pressure multiplied by 10. So 4.55 bar – 1 = 3.55 bar. At a depth of 35.5 meters the pressure is 3.55 bar.
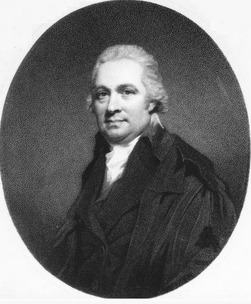
Because nitrogen occupies a large proportion of the breathing gas in terms of volume, it is also an important component in oxygen rebreathers. It is important because it can cause major problems if people use rebreathers without recognising a particular hazard. This danger consists of the fact that when an oxygen rebreather is put on, there is both oxygen and nitrogen in the counterlung. The diver will breathe in and out of the lung and will feel that he can breathe easily while the partial pressure of nitrogen will increase because the oxygen is used up and the exhaled carbon dioxide is absorbed by the scrubber. The diver will feel that he can breathe normally, but will be able to fall unconscious due to a lack of oxygen. If the carbon dioxide is not filtered out, the breathing stimulus is reinforced by a rising carbon dioxide level. If the carbon dioxide is not filtered out, the respiratory stimulus will be amplified by an increase in carbon dioxide. If the oxygen level drops below 0.1 bar partial pressure at the surface, unconsciousness will occur within 3 minutes. This is why a thorough training is necessary for the use of rebreathers. Here it will be learned that when using oxygen inrebreathers, flushing is necessary. This means breathing in from the counter-lung, breathing out to the environment and topping up with pure oxygen using the dosage button. This process must be repeated a number of times so that there is only pure oxygen in the counterlung.
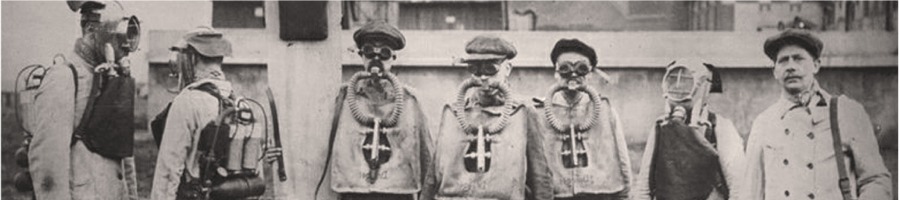
An oxygen rebreather can be used for diving, but the diving depth is very limited. Oxygen poisoning can occur from a partial pressure of 1.6 bar in water. This means that diving to a depth of 6 metres brings the risk of oxygen poisoning closer. Oxygen poisoning in a decompression tank can also occur, but humans can withstand higher oxygen partial pressures in a gaseous environment. Thus, decompression treatment with 2.0 bar is performed without any problems.
When diving with compressed air, a trained diver will be bothered by the nitrogen in his breathing gas at about 35-40 metres. Training and experience make a lot of difference in practice. Beginners are more likely to be affected by nitrogen than divers with a lot of experience at depth, due to various factors. Experience has shown that it is not training that makes divers experience nitrogen intoxication less, but getting used to the sensation. Strangely enough, some divers are able to dive to 65 metres on compressed air without much trouble (or so they think), while others experience the first symptoms at around 30 metres. Below is a video of Viktor Obtulovič that I found on youtube. Unfortunately I could not reach Viktor but because it was posted on youtube, I hope he recognizes the importance of this film and I hope he will allow me to show it to you.
So much has been written on the Internet that the Wikipedia page on this subject contains a great deal of information. However, it is absolutely necessary to undergo thorough diving training before exposing oneself to the risks described above.
After reading the section on oxygen and nitrogen, the reader may wonder how it is possible that divers with rebreathers dive much deeper than 40 metres. After all, they can dive 100 metres or deeper, whereas nitrogen forms a limit at around 35-40 metres and oxygen at around 1.6 bar partial pressure. This pressure of 1.6 bar is reached when diving with normal breathing air containing 21% oxygen (= 0.21 bar pO2) to a depth where the pressure is 1.6/0.21 = 7.6 bar, an actual depth of 66 metres. Below 66 metres, hyperoxia (oxygen intoxication) may occur, leading to immediate unconsciousness. The limit of nitrogen narcosis and oxygen poisoning can be overcome by reducing both partial pressures. In other words, less nitrogen and less oxygen in the mixture. This raises the question of what we then use to fill the resulting gap. This is usually done with helium. In this way, a mixture can be calculated with which one can dive to a depth of, for example, 90 metres. Suppose that a rebreather can keep the oxygen pressure at 1.3 bar by means of an electronic system, then the remaining gas must be composed of nitrogen and helium. As we do not want to come close to the nitrogen-nitrogen limit, we choose the nitrogen component at 3.0 bar at that depth. Since the ambient pressure at 90 metres is 10 bar, the helium component is 10 – 1.3 bar oxygen – 3.0 bar nitrogen = 5.7 bar helium. The gas that is breathed at this depth is then a mixture of 13% oxygen, 30% nitrogen and 57% helium. We call this a trimix mixture 13/57 or TX13/57. The rebreather must hold the 1.3 bar and if it breaks down we must be able to breathe a comparable gas from normal diving cylinders! We will explain more about this later.
Further exploration of the cause of decompression sickness:
Decompression sickness is the collective name for a wide range of symptoms and complaints, the exact cause and pathophysiology of which are not yet fully known. A number of names have been used to describe the syndrome over the years: caisson disease, aeropathy, bends, chokes, staggers and dysbaria. Today, decompression sickness is the most common name.
Further exploration of the cause of decompression sickness:
In 1670, R. Boyle described decompression sickness for the first time. Paul Bert demonstrated the effect of gas bubbles in the bloodstream on the functioning of the human body in 1878. Haldane developed the first decompression tables in 1908, which mathematically calculate the uptake and release of inert gas to bring divers and caissoners safely to the surface first at pressure and again after their work at depth. In the later diving and decompression models and tables, the body is seen as a combination of one or more different types of tissue, in which the absorption and release rates of gases are expressed in half-lives and some other physical constants.
Air consists of approximately 80 % nitrogen gas (N2). The partial pressure of nitrogen in body tissues is balanced by that in the lung alveoli. Nitrogen gas does not take part in the body’s metabolic processes and is therefore called “inert”. Henry’s gas law states that the dissolved mass of a gas in a liquid is determined by the partial pressure of that gas and its solubility coefficient.
During exposure to increased pressure, absorption of nitrogen gas occurs in the body. The rate of gas absorption is primarily determined by tissue perfusion. Tissues with good perfusion have a high absorption rate and are therefore called ‘fast’ tissues. On the other hand, “slow” tissues such as subcutaneous fatty tissue and cartilage, which are poorly supplied with blood, are. The diffusion speed of gases and their solubility in a tissue play a secondary role. Nitrogen dissolves five times as well in fatty tissue as in watery tissues such as muscle. The duration of exposure and the depth under water determine the amount of extra nitrogen absorbed in the body tissues. Perfusion and fat content of different tissues determine the variation and distribution of the total amount of extra nitrogen. When the diver or the caisson worker returns to the surface from the depth, the ambient pressure decreases again. This is known as decompression.
A reduction in the ambient pressure results in the release of the nitrogen stored in the tissues. If this nitrogen cannot leave the slow tissues quickly enough, a certain supersaturation with nitrogen will occur in the tissue concerned. If the supersaturation exceeds a certain limit, gas is produced by the passage of nitrogen from the dissolved form to the gaseous form: intravascular, interstitial and intracellular nitrogen bubbles are formed, which can cause obstruction, mechanical damage and haematological changes. These are the cause of the onset of decompression sickness.
Further read:
A recommendation: the best book I have read on decompression sickness that covers theory and physiology is Mark Powell’s book Deco for Divers. The book now comes in a second edition but the first may be easier to obtain. Version 1: ISBN 978-1-905492077 and
Version 2: 978-1-905492299
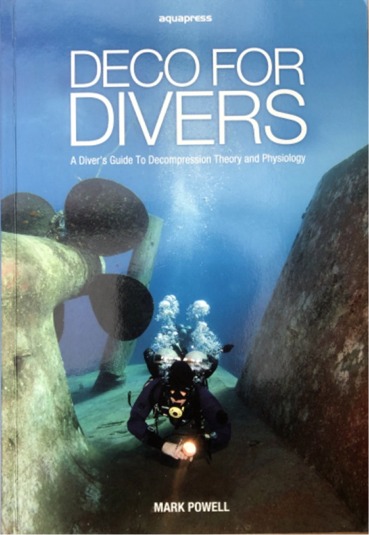